Deoxyribonucleic acid - DNA - serves as a carrier of hereditary information transmitted by living organisms to the next generations, and a matrix for the construction of proteins and various regulatory factors required by the body in the processes of growth and life. In this article, we will focus on what are the most common forms of DNA structure. We will also pay attention to how these forms are built and in what form DNA resides inside a living cell.
Levels of organization of the DNA molecule
There are four levels that determine the structure and morphology of this giant molecule:
- The primary level, or structure, is the order of the nucleotides in the chain.
- The secondary structure is the famous "double helix". It is this phrase that has settled down, although in fact such a structure resembles a screw.
- The tertiary structure is formed due to the fact that weak hydrogen bonds arise between individual sections of the double-stranded twisted strand of DNA,giving the molecule a complex spatial conformation.
- The quaternary structure is already a complex complex of DNA with some proteins and RNA. In this configuration, DNA is packaged into chromosomes in the cell nucleus.
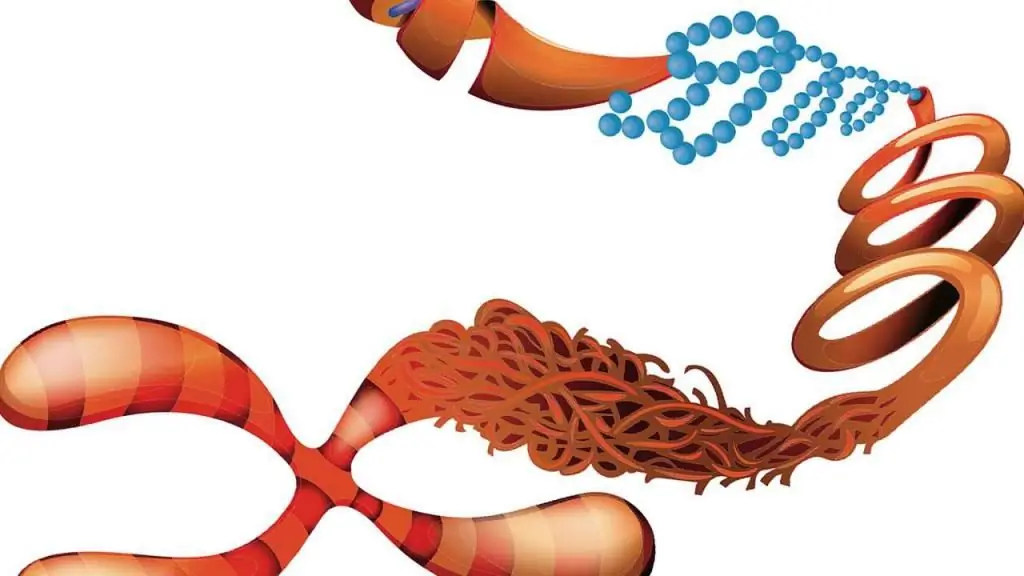
Primary Structure: Components of DNA
The blocks from which the macromolecule of deoxyribonucleic acid is built are nucleotides, which are compounds, each of which includes:
- nitrogenous base - adenine, guanine, thymine or cytosine. Adenine and guanine belong to the group of purine bases, cytosine and thymine belong to pyrimidine;
- five-carbon monosaccharide deoxyribose;
- Orthophosphoric acid residue.
In the formation of a polynucleotide chain, an important role is played by the order of groups formed by carbon atoms in a circular sugar molecule. The phosphate residue in the nucleotide is connected to the 5'-group (read "five primes") of deoxyribose, that is, to the fifth carbon atom. Chain extension occurs by attaching a phosphate residue of the next nucleotide to the free 3'-group of deoxyribose.
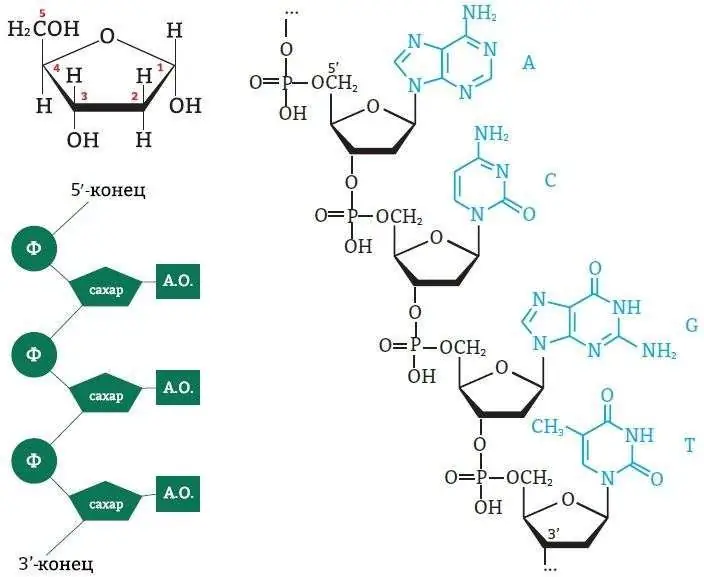
Thus, the primary structure of DNA in the form of a polynucleotide chain has 3'- and 5'-ends. This property of the DNA molecule is called polarity: the synthesis of a chain can only go in one direction.
Secondary structure formation
The next step in the structural organization of DNA is based on the principle of complementarity of nitrogenous bases - their ability to pair with each otherthrough hydrogen bonds. Complementarity - mutual correspondence - occurs because adenine and thymine form a double bond, and guanine and cytosine form a triple bond. Therefore, when forming a double chain, these bases stand opposite each other, forming the corresponding pairs.
Polynucleotide sequences are located in the secondary structure antiparallel. So, if one of the chains looks like 3' - AGGZATAA - 5', then the opposite will look like this: 3' - TTATGTST - 5'.
When a DNA molecule is formed, the doubled polynucleotide chain is twisted, and the concentration of s alts, water saturation, and the structure of the macromolecule itself determine what forms DNA can take at a given structural step. Several such forms are known, denoted by Latin letters A, B, C, D, E, Z.
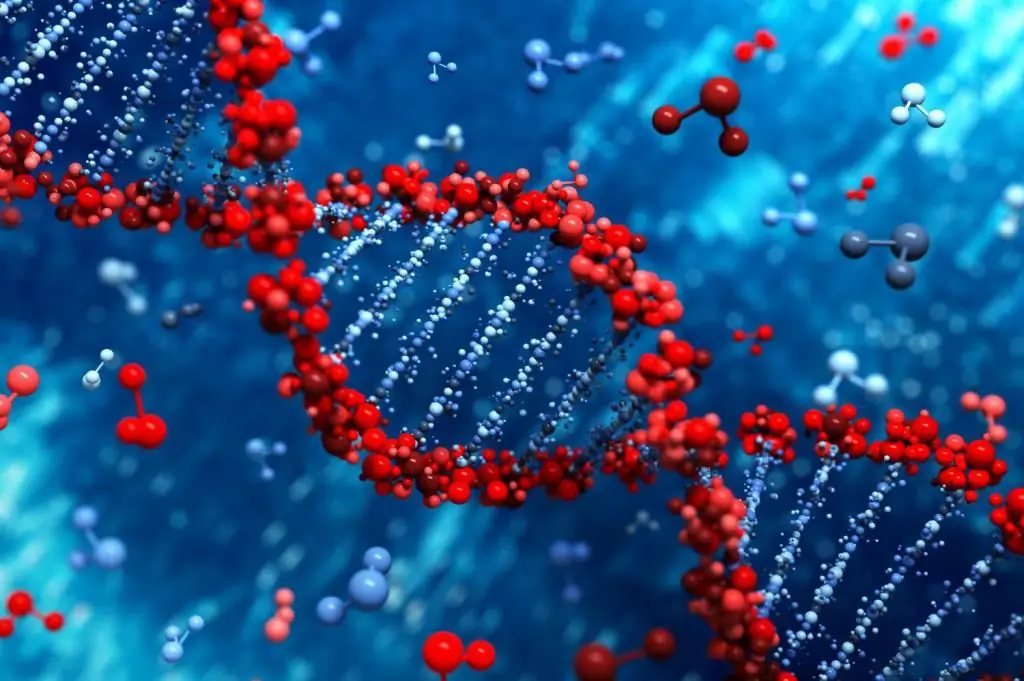
Configurations C, D and E are not found in wildlife and have only been observed in laboratory conditions. We will look at the main forms of DNA: the so-called canonical A and B, as well as the Z configuration.
A-DNA is a dry molecule
A-shape is a right hand screw with 11 complementary base pairs in each turn. Its diameter is 2.3 nm, and the length of one turn of the spiral is 2.5 nm. The planes formed by the paired bases have a slope of 20° with respect to the axis of the molecule. Neighboring nucleotides are compactly arranged in chains - there is only 0.23 nm between them.
This form of DNA occurs with low hydration and with an increased ionic concentration of sodium and potassium. It is typical forprocesses in which DNA forms a complex with RNA, since the latter is not able to take other forms. In addition, the A-form is highly resistant to ultraviolet radiation. In this configuration, deoxyribonucleic acid is found in fungal spores.
Wet B-DNA
With a low s alt content and a high degree of hydration, that is, under normal physiological conditions, DNA assumes its main form B. Natural molecules exist, as a rule, in the B-form. It is she who underlies the classical Watson-Crick model and is most often depicted in illustrations.
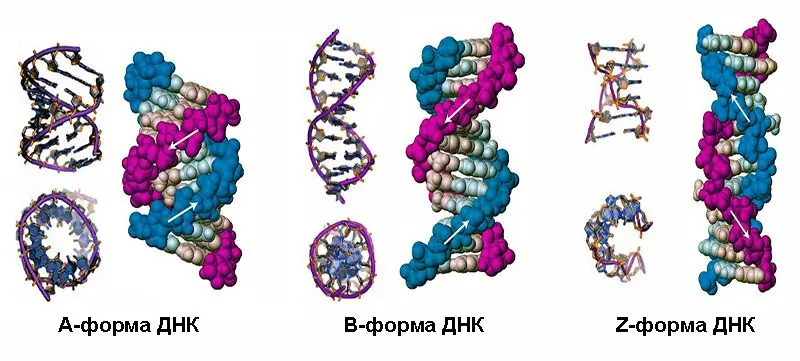
This form (it is also right-handed) is characterized by less compact placement of nucleotides (0.33 nm) and a large screw pitch (3.3 nm). One turn contains 10.5 base pairs, the rotation of each of them relative to the previous one is about 36 °. The planes of the pairs are almost perpendicular to the axis of the "double helix". The diameter of such a double chain is smaller than that of the A-form - it reaches only 2 nm.
Non-canonical Z-DNA
Unlike canonical DNA, the Z-type molecule is a left-handed screw. It is the thinnest of all, having a diameter of only 1.8 nm. Its coils, 4.5 nm long, seem to be elongated; this form of DNA contains 12 paired bases per turn. The distance between adjacent nucleotides is also quite large - 0.38 nm. So the Z-shape has the least twist.
It is formed from a B-type configuration in those areas where purineand pyrimidine bases, with a change in the content of ions in solution. The formation of Z-DNA is associated with biological activity and is a very short-term process. This form is unstable, which creates difficulties in the study of its functions. So far, they are not exactly clear.
DNA replication and its structure
Both the primary and secondary structures of DNA arise during a phenomenon called replication - the formation of two identical "double helixes" from the parent macromolecule. During replication, the original molecule unwinds, and complementary bases build up on the released single chains. Since the DNA halves are antiparallel, this process proceeds on them in different directions: in relation to the parent chains from the 3'-end to the 5'-end, that is, new chains grow in the direction 5' → 3'. The leading strand is synthesized continuously towards the replication fork; on the lagging strand, synthesis is performed from the fork in separate sections (Okazaki fragments), which are then sewn together by a special enzyme, DNA ligase.
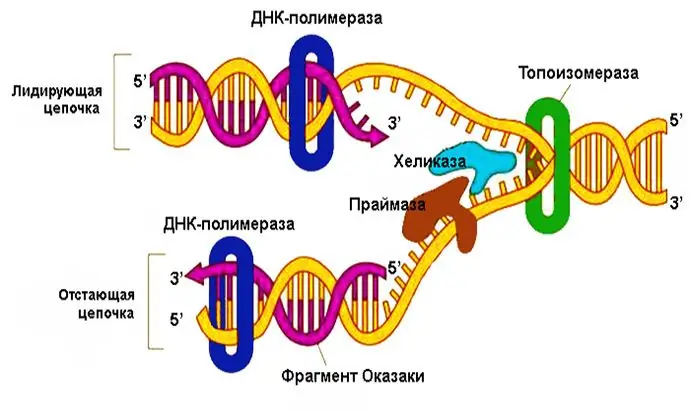
While the synthesis continues, the already formed ends of the daughter molecules undergo helical twisting. Then, before replication is complete, the newborn molecules begin to form a tertiary structure in a process called supercoiling.
Super Twisted Molecule
The supercoiled form of DNA occurs when a double-stranded molecule makes an extra twist. It can be clockwise (positive) oragainst (in this case one speaks of negative supercoiling). The DNA of most organisms is supercoiled negatively, that is, against the main turns of the “double helix.”
As a result of the formation of additional loops - supercoils - DNA acquires a complex spatial configuration. In eukaryotic cells, this process occurs with the formation of complexes in which DNA coils negatively around histone protein complexes and takes the form of a thread with nucleosome beads. Free sections of the thread are called linkers. Non-histone proteins and inorganic compounds also take part in maintaining the supercoiled shape of the DNA molecule. This is how chromatin is formed - the substance of chromosomes.
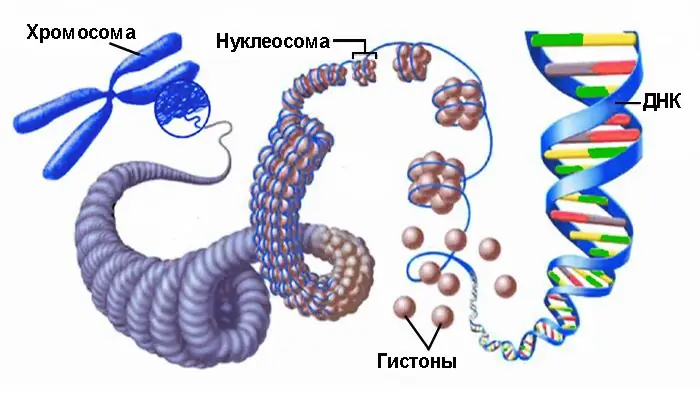
Chromatin strands with nucleosome beads are capable of further complicating morphology in a process called chromatin condensation.
Final compactization of DNA
In the nucleus, the shape of the deoxyribonucleic acid macromolecule becomes extremely complex, compacting in several steps.
- Firstly, the filament is coiled into a special solenoid-type structure - a chromatin fibril 30 nm thick. At this level, DNA folds and shortens its length by 6-10 times.
- Further, the fibril forms zigzag loops with the help of specific scaffold proteins, which reduces the linear size of DNA already by 20-30 times.
- At the next level, densely packed loop domains are formed, most often having a shape conventionally called "lamp brush". They attach to the intranuclear proteinmatrix. The thickness of such structures is already 700 nm, while DNA is shortened by approximately 200 times.
- The last level of morphological organization is chromosomal. The loop domains are compacted to such an extent that a total shortening of 10,000 times is achieved. If the length of the stretched molecule is about 5 cm, then after packing into chromosomes it decreases to 5 microns.
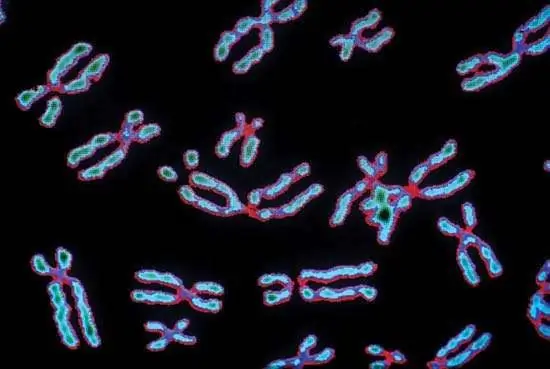
The highest level of complication of the form of DNA reaches in the state of metaphase of mitosis. It is then that it acquires a characteristic appearance - two chromatids connected by a constriction-centromere, which ensures the divergence of chromatids in the process of division. Interphase DNA is organized down to the domain level and is distributed in the cell nucleus in no particular order. Thus, we see that the morphology of DNA is closely related to the various phases of its existence and reflects the features of the functioning of this most important molecule for life.