Thermodynamics is an important branch of physics. We can safely say that its achievements have led to the emergence of the technological era and have largely determined the course of human history over the past 300 years. The article discusses the first, second and third laws of thermodynamics and their application in practice.
What is thermodynamics?
Before formulating the laws of thermodynamics, let's figure out what this section of physics does.
The word "thermodynamics" is of Greek origin and means "movement due to heat". That is, this branch of physics is engaged in the study of any processes, as a result of which thermal energy is converted into mechanical motion and vice versa.
The basic laws of thermodynamics were formulated in the middle of the 19th century. The science of "movement and heat" considers the behavior of the entire system as a whole, studying the change in its macroscopic parameters - temperature, pressure and volume, and not paying attention to its microscopic structure. Moreover, the first of them plays a fundamental role in the formulation of lawsthermodynamics in physics. It is curious to note that they are derived solely from experimental observations.
The concept of a thermodynamic system
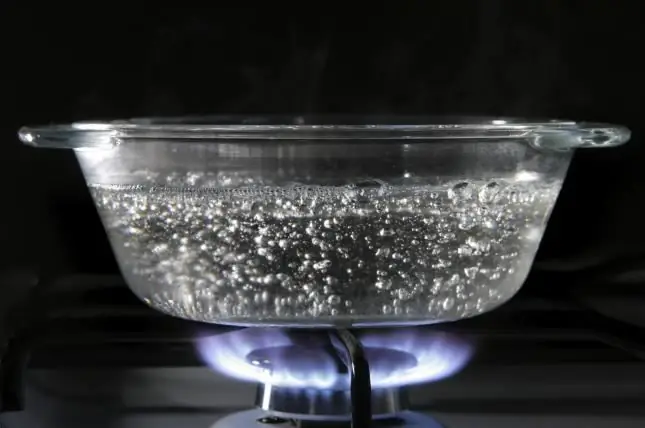
It means any group of atoms, molecules or other elements that are considered as a whole. All three laws are formulated for the so-called thermodynamic system. Examples are: Earth's atmosphere, any living organism, gas mixture in an internal combustion engine, etc.
All systems in thermodynamics belong to one of three types:
- Open. They exchange both heat and matter with the environment. For example, if food is cooked in a pot on an open fire, then this is a vivid example of an open system, since the pot receives energy from the external environment (fire), while it itself radiates energy in the form of heat, and water also evaporates from it (metabolism).
- Closed. In such systems there is no exchange of matter with the environment, although the exchange of energy occurs. Returning to the previous case: if you cover the pot with a lid, you can get a closed type system.
- Isolated. This is a kind of thermodynamic systems that do not exchange matter or energy with the surrounding space. An example is a thermos containing hot tea.
Thermodynamic temperature
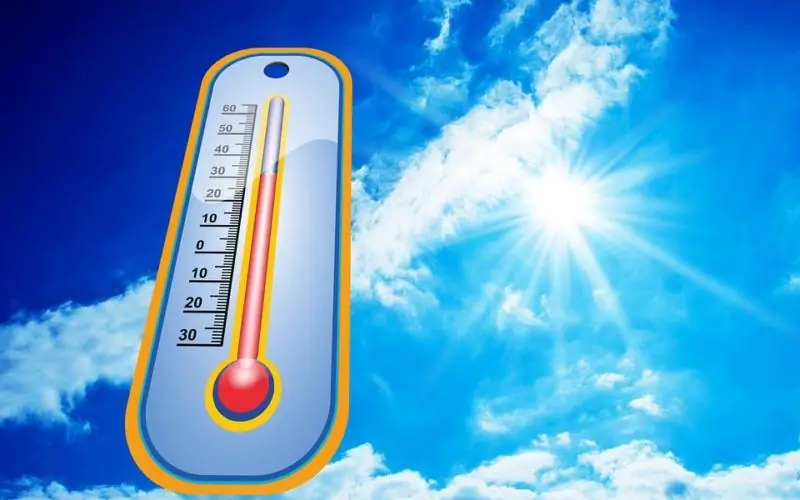
This concept means the kinetic energy of the particles that form the surrounding bodies, which reflects the speedrandom movement of particles. The larger it is, the higher the temperature. Accordingly, by reducing the kinetic energy of the system, we cool it.
This concept means the kinetic energy of the particles that form the surrounding bodies, which reflects the speed of the chaotic movement of particles. The larger it is, the higher the temperature. Accordingly, by reducing the kinetic energy of the system, we cool it.
Thermodynamic temperature is expressed in SI (International System of Units) in Kelvin (in honor of the British scientist William Kelvin, who first proposed this scale). Understanding the first, second and third laws of thermodynamics is impossible without a definition of temperature.
A division of one degree on the Kelvin scale also corresponds to one degree Celsius. The conversion between these units is carried out according to the formula: TK =TC + 273, 15, where TK and TC - temperatures in kelvins and degrees Celsius respectively.
The peculiarity of the Kelvin scale is that it does not have negative values. Zero in it (TC=-273, 15 oC) corresponds to the state when the thermal motion of the particles of the system is completely absent, they are, as it were, "frozen ".
Conservation of energy and the 1st law of thermodynamics
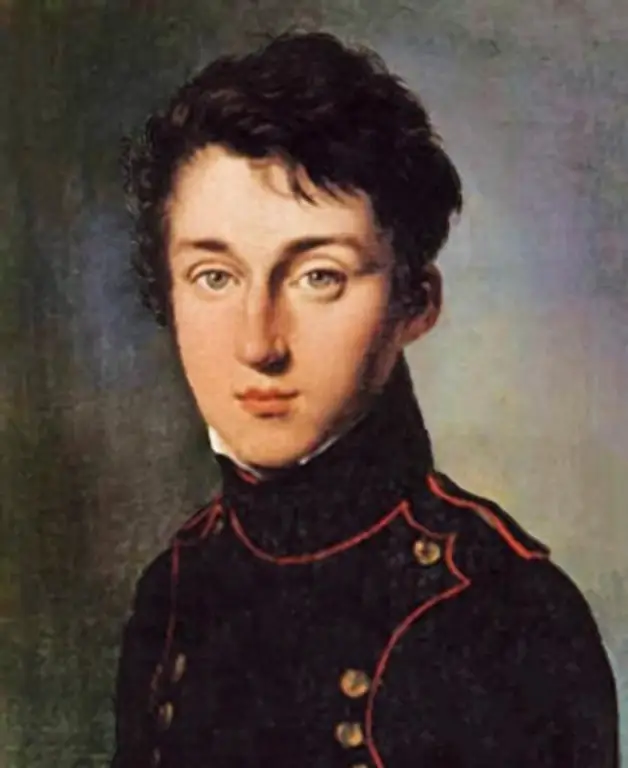
In 1824, Nicolas Léonard Sadi Carnot, a French engineer and physicist, made a bold suggestion that not only led to the development of physics, but also became a major step in the improvement of technology. Hiscan be formulated as follows: "Energy cannot be created or destroyed, it can only be transferred from one state to another."
In fact, Sadi Carnot's phrase postulates the law of conservation of energy, which formed the basis of the 1st law of thermodynamics: "Whenever a system receives energy from the outside, it converts it into other forms, the main of which are thermal and mechanical."
The mathematical formula for the 1st law is written as follows:
Q=ΔU + A, here Q is the amount of heat transferred by the environment to the system, ΔU is the change in the internal energy of this system, A is the perfect mechanical work.
Adiabatic processes
A good example of them is the movement of air masses along mountain slopes. Such masses are huge (kilometers or more), and air is an excellent heat insulator. The noted properties allow us to consider any processes with air masses that occur within a short time as adiabatic. When air rises up a mountain slope, its pressure drops, it expands, that is, it performs mechanical work, and, as a result, it cools. On the contrary, the downward movement of the air mass is accompanied by an increase in pressure in it, it compresses and, due to this, becomes very hot.
The application of the law of thermodynamics, which was discussed in the previous subheading, is most easily demonstrated using the example of an adiabatic process.
According to the definition, as a result of it there is no exchange of energy withenvironment, that is, in the equation above, Q=0. This leads to the following expression: ΔU=-A. The minus sign here means that the system performs mechanical work by reducing its own internal energy. It should be recalled that the internal energy is directly dependent on the temperature of the system.
Direction of thermal processes
This issue deals with the 2nd law of thermodynamics. Surely everyone noticed that if you bring two objects with different temperatures into contact, then the cold one will always heat up, and the hot one will cool down. Note that the reverse process can occur within the framework of the first law of thermodynamics, but it is never implemented in practice.
The reason for the irreversibility of this process (and all known processes in the Universe) is the transition of the system to a more probable state. In the considered example with the contact of two bodies of different temperatures, the most probable state will be the one in which all particles of the system will have the same kinetic energy.
The second law of thermodynamics can be formulated as follows: "Heat can never spontaneously be transferred from a cold body to a hot one." If we introduce the concept of entropy as a measure of disorder, then it can be represented as follows: "Any thermodynamic process proceeds with an increase in entropy".
Heat engine
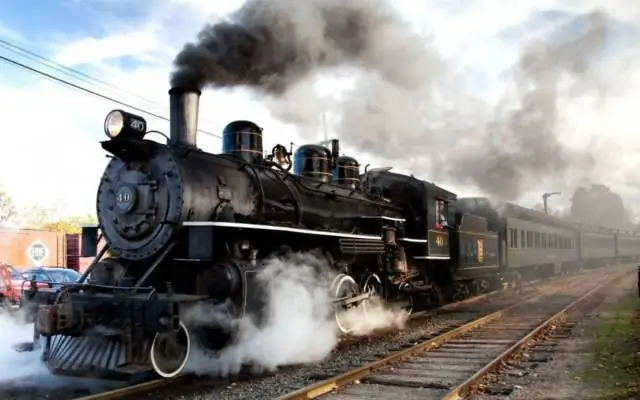
This term is understood as a system that, due to the supply of external energy to it, can perform mechanical work. Firstheat engines were steam engines and were invented at the end of the 17th century.
The second law of thermodynamics plays a decisive role in determining their effectiveness. Sadi Carnot also established that the maximum efficiency of this device is: Efficiency=(T2 - T1)/T2 , here T2 and T1 are heater and refrigerator temperatures. Mechanical work can only be done when there is a flow of heat from a hot body to a cold one, and this flow cannot be 100% converted into useful energy.
The figure below shows the principle of operation of a heat engine (Qabs - heat transferred to the machine, Qced - heat loss, W - useful work, P and V - pressure and volume of gas in the piston).
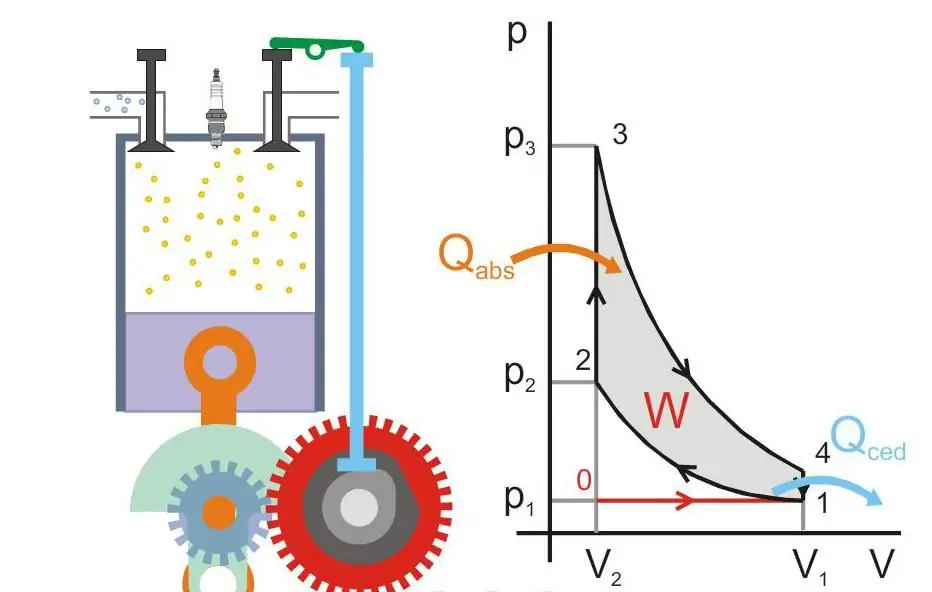
Absolute zero and Nernst's postulate
Finally, let's move on to the consideration of the third law of thermodynamics. It is also called the Nernst postulate (the name of the German physicist who first formulated it at the beginning of the 20th century). The law says: "Absolute zero cannot be reached with a finite number of processes." That is, it is impossible in any way to completely "freeze" the molecules and atoms of a substance. The reason for this is the constant existing heat exchange with the environment.
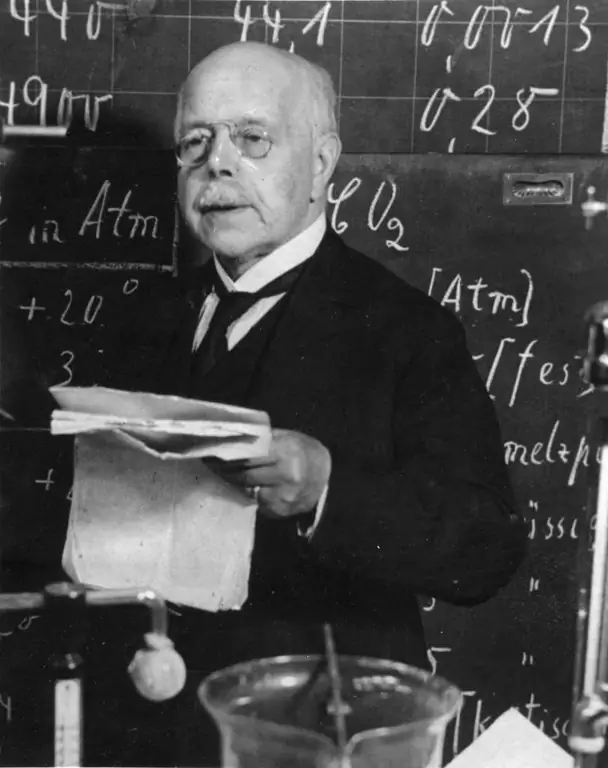
One useful conclusion drawn from the third law of thermodynamics is that entropy decreases as one moves towards absolute zero. This means that the system tends to organize itself. This fact canuse, for example, to transfer paramagnets to a ferromagnetic state when cooled.
It is interesting to note that the lowest temperature that has been reached so far is 5·10−10 K (2003, MIT laboratory, USA).