What is the working principle of X-ray laser? Because of the high gain in the generation medium, the short upper state lifetimes (1-100 ps), and the problems associated with building mirrors that can reflect the beams, these lasers typically operate without mirrors. The X-ray beam is generated by a single pass through the gain medium. The emitted radiation based on the amplified spontaneous beam has a relatively low spatial coherence. Read the article to the end and you will understand that this is an X-ray laser. This device is very practical and unique in its structure.
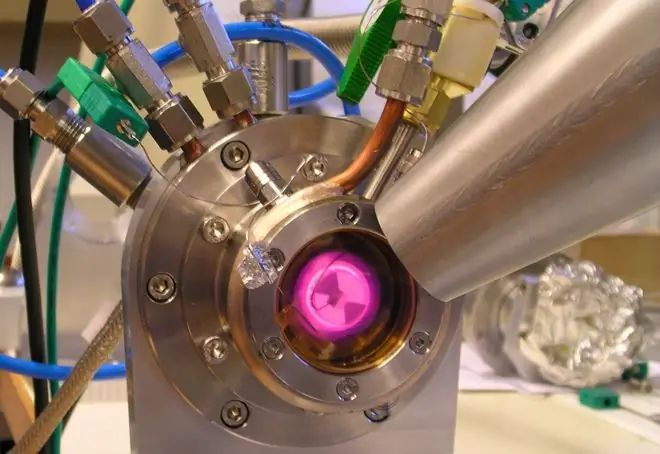
Kernels in the mechanism structure
Since conventional laser transitions between visible and electronic or vibrational states correspond to energies up to 10 eV, different active media are needed for X-ray lasers. Again, various active charged nuclei can be used for this.
Weapons
Between 1978 and 1988 in the Excalibur projectThe US military attempted to develop a nuclear explosive X-ray laser for missile defense as part of the Star Wars Strategic Defense Initiative (SDI). The project, however, turned out to be too expensive, dragged on, and was eventually shelved.
Plasma media inside a laser
The most commonly used media include highly ionized plasma created in a capillary discharge or when a linearly focused optical pulse hits a solid target. According to the Saha ionization equation, the most stable electron configurations are neon, with 10 electrons remaining, and nickel-like, with 28 electrons. Electron transitions in highly ionized plasmas typically correspond to energies on the order of hundreds of electron volts (eV).
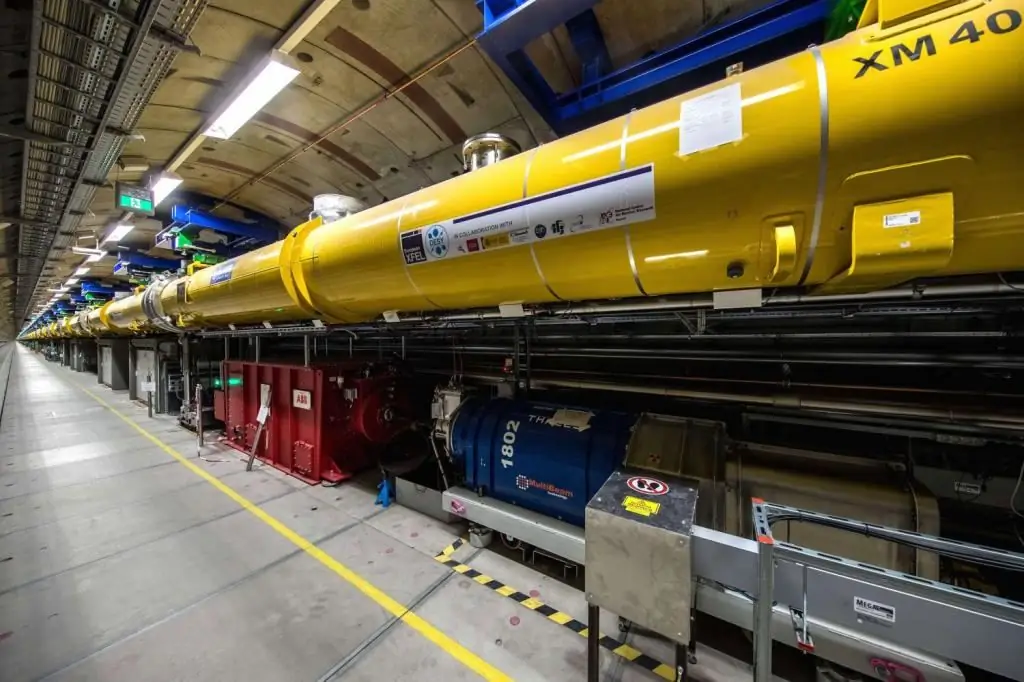
An alternative amplifying medium is the relativistic electron beam of an X-ray free electron laser, which uses stimulated Compton scattering instead of standard radiation.
Application
Coherent X-ray applications include coherent diffraction imaging, dense plasma (opaque to visible radiation), X-ray microscopy, phase-resolved medical imaging, material surface examination, and weaponization.
Lighter version of the laser can be used for ablative laser movement.
X-ray laser: how it works
How do lasers work? Due to the fact that the photonhits an atom with a certain energy, you can make the atom emit a photon with that energy in a process called stimulated emission. By repeating this process on a large scale, you will get a chain reaction that results in a laser. However, some quantum knots cause this process to stop, as a photon is sometimes absorbed without being emitted at all. But to ensure the best chance, photon energy levels are increased and mirrors are placed parallel to the light path to help the scattered photons come back into play. And at high energies of X-rays, special physical laws are found that are inherent in this particular phenomenon.
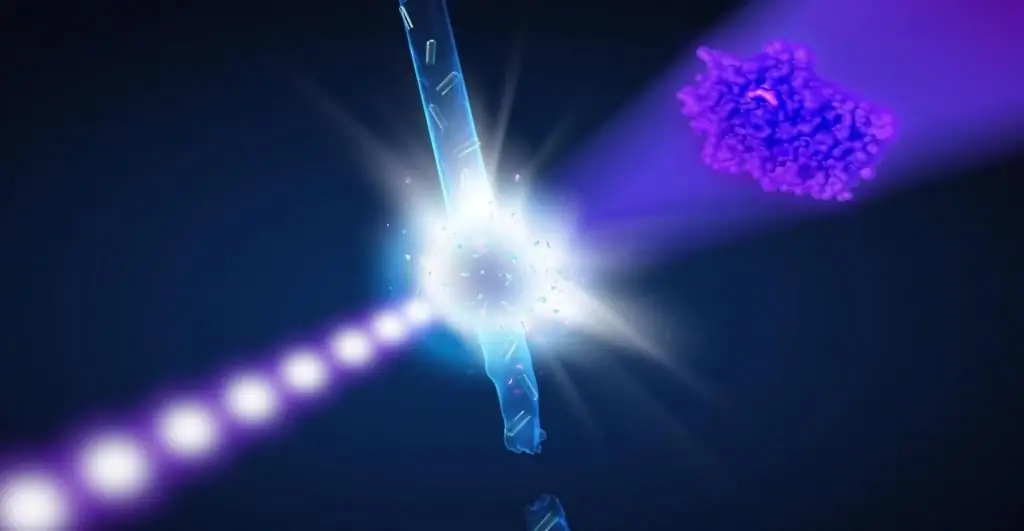
History
In the early 1970s, the X-ray laser seemed out of reach, as most lasers of the day peaked at 110 nm, well below the largest X-rays. This was because the amount of energy required to produce the stimulated material was so high that it had to be delivered in a fast pulse, further complicating the reflectivity needed to create a high-power laser. Therefore, scientists looked at the plasma, because it looked like a good conducting medium. A team of scientists in 1972 claimed they had finally achieved the use of plasma in the creation of lasers, but when they tried to reproduce their previous results, they failed for some reason.
In the 1980s, a major player from the world joined the research teamScience - Livermore. Scientists meanwhile have been making small but important strides for years, but after the Defense Advanced Research Projects Agency (DARPA) stopped paying for X-ray research, Livermore became the leader of the scientific team. He led the development of several types of lasers, including those based on fusion. Their nuclear weapons program was promising, because the high energy indicators that scientists achieved during this program hinted at the possibility of creating a high-quality pulsed mechanism that would be useful in the construction of an X-ray free electron laser.
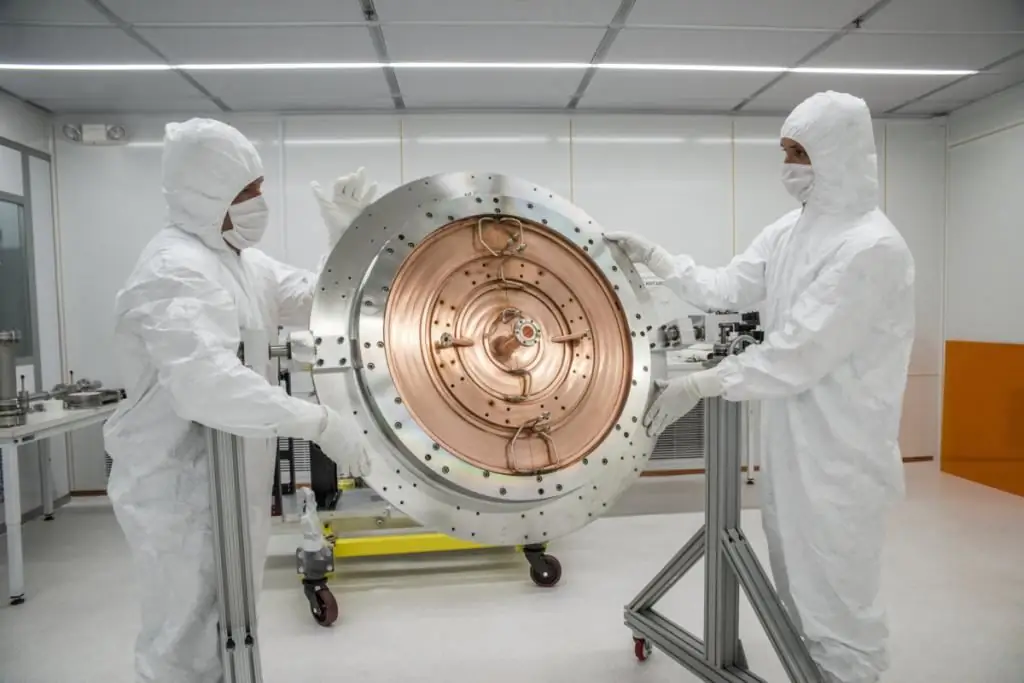
The project was gradually approaching completion. Scientists George Chaplin and Lowell Wood first explored fusion technology for X-ray lasers in the 1970s and then switched to a nuclear option. Together they developed such a mechanism and were ready for testing on September 13, 1978, but equipment failure cut it short. But perhaps it was for the best. Peter Hagelstein created a different approach after studying the previous mechanism, and on November 14, 1980, two experiments proved that the prototype X-ray laser worked.
Star Wars Project
Very soon, the US Department of Defense became interested in the project. Yes, using the power of a nuclear weapon in a focused beam is too dangerous, but that power could be used to destroy intercontinental ballistic missiles (ICBMs) in the air. It would be most convenient to use a similar mechanism on the near-Earthorbit. The whole world knows this program called Star Wars. However, the project to use the X-ray laser as a weapon never came to fruition.
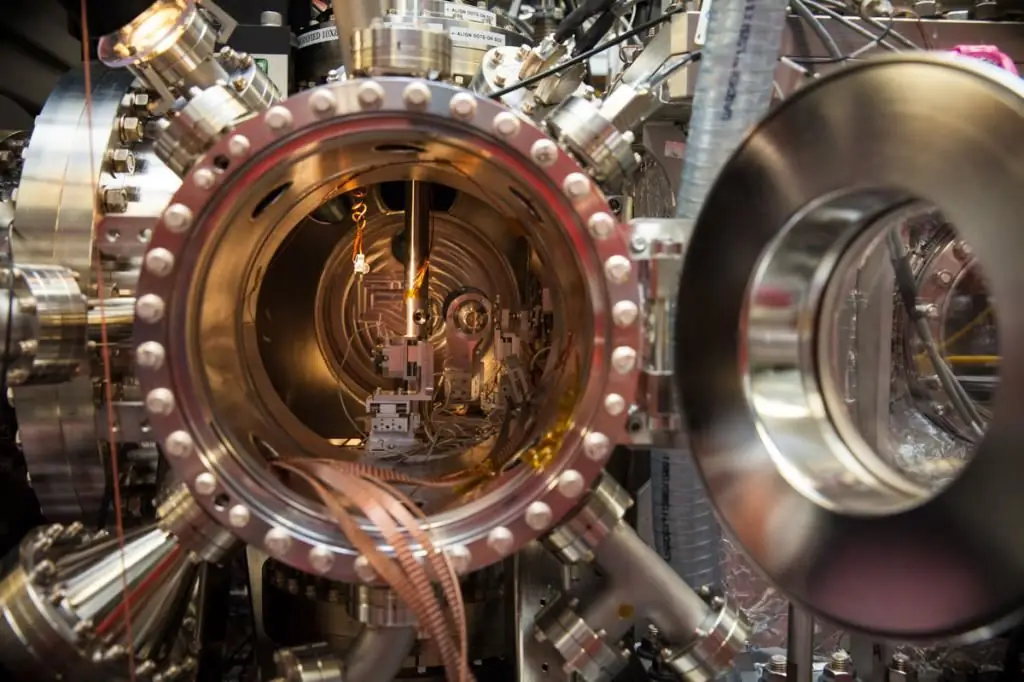
The February 23, 1981 issue of Aviation Week and Space Engineering reports the results of the first tests of the project, including a laser beam that reached 1.4 nanometers and hit 50 different targets.
Tests dated March 26, 1983 yielded nothing due to sensor failure. However, the following tests on December 16, 1983 demonstrated its true capabilities.
Further fate of the project
Hagelstein envisioned a two-step process in which a laser would create a plasma that would release charged photons that would collide with electrons in another material and cause X-rays to be emitted. Several setups were tried, but in the end ion manipulation proved to be the best solution. The plasma removed the electrons until only 10 inner ones were left, where the photons then charged them up to the 3p state, thus releasing the "soft" beam. An experiment on July 13, 1984 proved that this was more than theory when a spectrometer measured strong emissions at 20.6 and 20.9 nanometers of selenium (a neon-like ion). Then the first laboratory (not military) X-ray laser appeared with the name Novette.
The fate of Novette
This laser was designed by Jim Dunn and had physical aspects verified by Al Osterheld and Slava Shlyaptsev. Using fast(near nanosecond) pulse of high-energy light that charged the particles to release X-rays, Novett also used glass amplifiers, which improve efficiency but also heat up quickly, meaning it can only run 6 times a day between cooldowns. But some work has shown that it can fire a picosecond pulse while the compression returns to a nanosecond pulse. Otherwise, the glass amplifier will be destroyed. It is important to note that Novette and other "desktop" X-ray lasers produce "soft" X-ray beams, which have a longer wavelength, which prevents the beam from passing through many materials, but gives insight into alloys and plasma, since it easily shines through them.
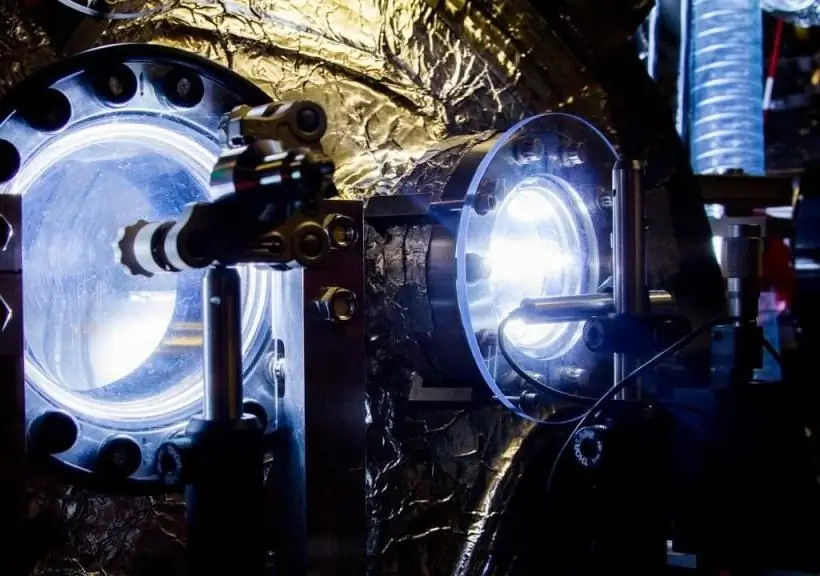
Other uses and features of operation
So what can this laser be used for? It has previously been noted that a shorter wavelength can make it easier to examine some materials, but this is not the only application. When a target is hit by an impulse, it is simply destroyed into atomic particles, and the temperature at the same time reaches millions of degrees in just a trillionth of a second. And if this temperature is enough, the laser will cause the electrons to peel off from the inside. This is because the lowest level of electron orbitals implies the presence of at least two electrons, which are ejected from the energy generated by X-rays.
The time it takes for an atom tohas lost all its electrons, is on the order of a few femtoseconds. The resulting core does not linger for long and rapidly transitions into a plasma state known as "warm dense matter", which is mostly found in nuclear reactors and the cores of large planets. By experimenting with the laser, we can get an idea of both processes, which are different forms of nuclear fusion.
The use of the X-ray laser is truly universal. Another useful feature of these X-rays is their use with synchrotrons or particles accelerating along the entire path of the accelerator. Based on how much energy it takes to make this path, the particles can emit radiation. For example, electrons, when excited, emit X-rays, which have a wavelength about the size of an atom. Then we could study the properties of these atoms through interaction with X-rays. In addition, we can change the energy of the electrons and obtain different wavelengths of X-rays, achieving greater depth of analysis.
However, it is very difficult to create an X-ray laser with your own hands. Its structure is extremely complex even from the point of view of experienced physicists.
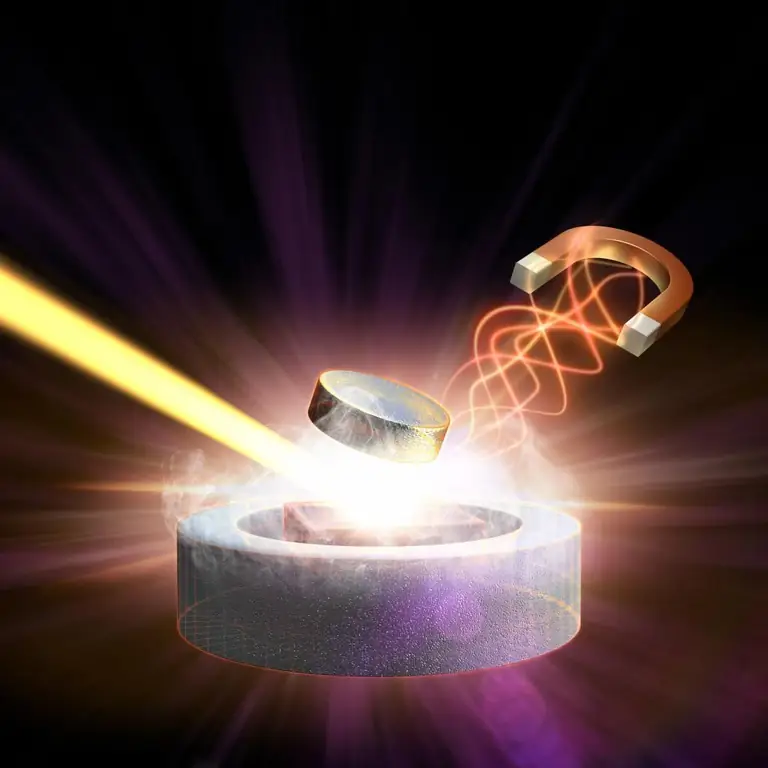
In biology
Even biologists have been able to benefit from x-ray lasers (nuclear pumped). Their radiation can help reveal aspects of photosynthesis previously unknown to science. They capture subtle changes in plant leaves. Long wavelengths of soft X-ray laser beams allow you to explore without destroying everything thattakes place inside the plant. The nanocrystal injector triggers photocell I, the protein key to photosynthesis needed to activate it. This is intercepted by a laser beam of X-rays, which causes the crystal to literally explode.
If the above experiments continue to be successful, people will be able to unravel the mysteries of nature, and artificial photosynthesis may become a reality. It will also raise the question of the possibility of more efficient use of solar energy, provoking the emergence of scientific projects for many years to come.
Magnets
How about an electronic magnet? The scientists found that when they had xenon atoms and iodine-limited molecules hit by a high-power X-ray, the atoms threw off their inner electrons, creating a void between the nucleus and the outermost electrons. Attractive forces set these electrons in motion. Normally this shouldn't happen, but due to the suddenness of the dropping of electrons, an overly "charged" situation occurs at the atomic level. Scientists think the laser could be used in image processing.
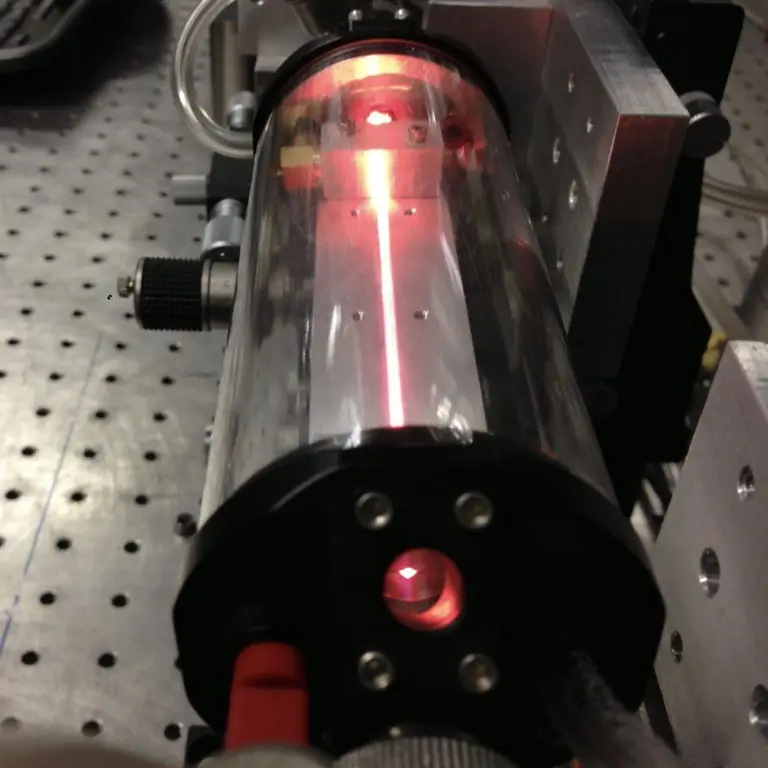
Giant X-ray laser Xfel
Hosted at the US National Accelerator Laboratory, specifically at the linac, this 3,500-foot laser uses several ingenious devices to hit targets with hard X-rays. Here are some of the components of one of the most powerful lasers (abbreviations and anglicisms stand for the components of the mechanism):
- Drive Laser - createsan ultraviolet pulse that removes electrons from the cathode. Emits electrons up to an energy level of 12 billion eW by manipulating the electric field. Also inside the movement there is an S-shaped accelerator called Bunch Compressor 1.
- Bunch Compressor 2 - same concept as Bunch 1 but longer S-shaped structure, increased due to higher energies.
- Transport Hall - allows you to make sure that the electrons are suitable for focusing pulses using magnetic fields.
- Undulator Hall - Consists of magnets that cause electrons to move back and forth, thereby generating high-energy x-rays.
- Beam Dump is a magnet that removes electrons but lets X-rays through without moving.
- LCLS Experimental Station is a special chamber in which the laser is fixed and which is the main space for experiments related to it. The beams generated by this device create 120 pulses per second, with each pulse lasting 1/10000000000 of a second.
- Capillary plasma-discharge medium. In this setup, a capillary several centimeters long, made of a stable material (eg alumina), limits a high-precision, sub-microsecond electrical pulse in a low-pressure gas. The Lorentz force causes further compression of the plasma discharge. In addition, a pre-ionization electrical or optical pulse is often used. An example is a capillary neon-like Ar8 + laser (which generates radiation at 47nm).
- Target medium of a solid slab - after being hit by an optical pulse, the target emits a highly excited plasma. Again, a longer "prepulse" is often used to create the plasma, and a second, shorter and more energetic pulse is used to further heat the plasma. For short lifetimes, a momentum shift may be required. The refractive index gradient of the plasma causes the amplified pulse to bend away from the target surface, since at frequencies above resonance the refractive index decreases with the density of the matter. This can be compensated for by using multiple targets in a burst, as in the European X-ray free electron laser.
- Plasma excited by an optical field - at optical densities high enough to effectively tunnel electrons or even to suppress a potential barrier (> 1016 W / cm2), it is possible to strongly ionize a gas without contact with a capillary or target. Typically a collinear setting is used to synchronize the pulses.
In general, the structure of this mechanism is similar to the European X-ray free electron laser.