This article contains a description of such a thing as X-ray diffraction. The physical basis of this phenomenon and its applications are explained here.
Technologies for creating new materials
Innovation, nanotechnology is the trend of the modern world. The news is full of reports of new revolutionary materials. But few people think about what a huge research apparatus scientists need to create at least a small improvement in existing technologies. One of the fundamental phenomena that help people do this is X-ray diffraction.
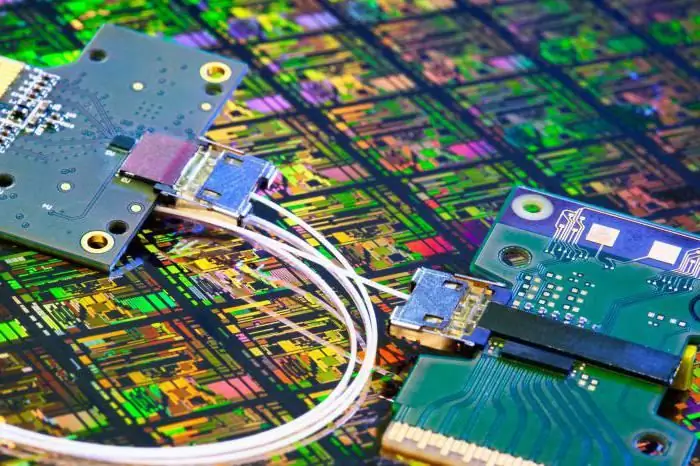
Electromagnetic radiation
First you need to explain what electromagnetic radiation is. Any moving charged body generates an electromagnetic field around itself. These fields pervade everything around, even the vacuum of deep space is not free from them. If in such a field there are periodic perturbations that can propagate in space, they are called electromagnetic radiation. To describe it, concepts such as wavelength, frequency and its energy are used. What is energy is intuitive, and the wavelength is the distance betweenidentical phases (for example, between two adjacent maxima). The higher the wavelength (and, accordingly, the frequency), the lower its energy. Recall that these concepts are necessary to describe what X-ray diffraction is concisely and succinctly.
Electromagnetic spectrum
All variety of electromagnetic rays fit on a special scale. Depending on the wavelength, they distinguish (from the longest to the shortest):
- radio waves;
- terahertz waves;
- infrared waves;
- visible waves;
- ultraviolet waves;
- X-ray waves;
- gamma radiation.
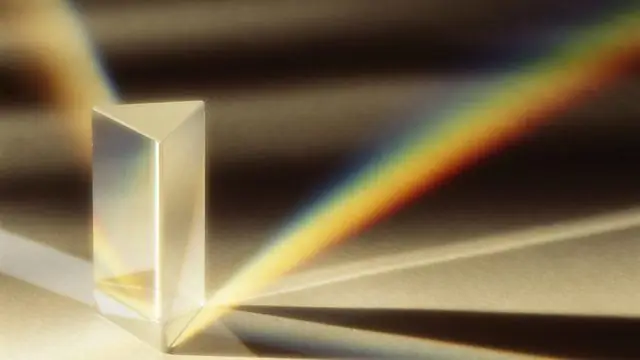
Thus, the radiation we are interested in has a very short wavelength and the highest energies (which is why it is sometimes called hard). Therefore, we are getting closer to describing what X-ray diffraction is.
The origin of X-rays
The higher the radiation energy, the more difficult it is to obtain it artificially. Having made a fire, a person receives a lot of infrared radiation, because it is it that transfers heat. But for the diffraction of X-rays by spatial structures to occur, a lot of effort must be made. So, this kind of electromagnetic radiation is released when an electron is knocked out of the shell of an atom, which is close to the nucleus. The electrons located above tend to fill the resulting hole, their transitions and give X-ray photons. Also, during sharp deceleration of charged particles with mass (for example,electrons), these high-energy beams are produced. Thus, the diffraction of X-rays on a crystal lattice is accompanied by the expenditure of a fairly large amount of energy.
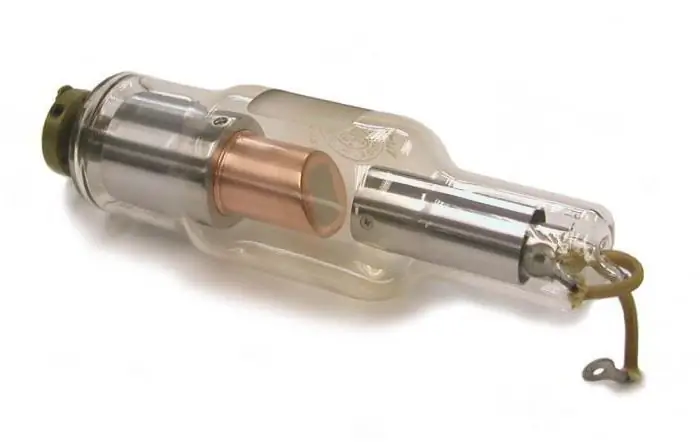
On an industrial scale, this radiation is obtained as follows:
- The cathode emits a high-energy electron.
- Electron collides with the material of the anode.
- The electron decelerates sharply (while emitting X-rays).
- In another case, the decelerating particle knocks an electron out of the atom's low orbit from the anode material, which also generates X-rays.
It is also necessary to understand that, like any other electromagnetic radiation, X-rays have their own spectrum. This radiation itself is used quite widely. Everyone knows that a broken bone or a mass in the lungs is looked for with the help of x-rays.
Structure of a crystalline substance
Now we come close to what the X-ray diffraction method is. To do this, it is necessary to explain how a solid body is arranged. In science, a solid body is called any substance in a crystalline state. Wood, clay or glass are solid, but they lack the main thing: a periodic structure. But crystals have this amazing property. The very name of this phenomenon contains its essence. First you need to understand that the atoms in the crystal are fixed rigidly. The bonds between them have some degree of elasticity, but they are too strong for atoms to move around inside.gratings. Such episodes are possible, but with a very strong external influence. For example, if a metal crystal is bent, point defects of various types are formed in it: in some places, the atom leaves its place, forming a vacancy, in others, it moves to the wrong positions, forming an interstitial defect. At the bend, the crystal loses its slender crystalline structure, becomes very defective, loose. Therefore, it is better not to use a paper clip that has been unbent once, as the metal has lost its properties.
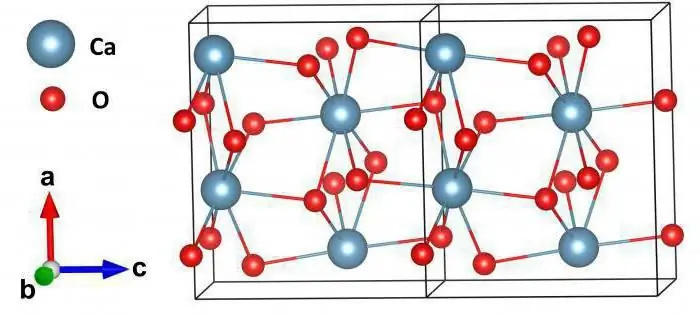
If the atoms are fixed rigidly, they can no longer be randomly arranged relative to each other, as in liquids. They must organize themselves in such a way as to minimize the energy of their interaction. Thus, the atoms line up in a lattice. In each lattice there is a minimum set of atoms arranged in a special way in space - this is the elementary cell of the crystal. If we broadcast it entirely, that is, combine the edges with each other, shifting in any direction, we will get the entire crystal. However, it is worth remembering that this is a model. Any real crystal has defects, and it is almost impossible to achieve an absolutely accurate translation. Modern silicon memory cells are close to ideal crystals. However, obtaining them requires incredible amounts of energy and other resources. In the laboratory, scientists obtain perfect structures of various kinds, but, as a rule, the costs of their creation are too high. But we will assume that all crystals are ideal: in anydirection, the same atoms will be located at the same distances from each other. This structure is called a crystal lattice.
Study of crystal structure
It is thanks to this fact that X-ray diffraction on crystals is possible. The periodic structure of crystals creates certain planes in them, in which there are more atoms than in other directions. Sometimes these planes are set by the symmetry of the crystal lattice, sometimes by the mutual arrangement of atoms. Each plane is assigned its own designation. The distances between the planes are very small: on the order of several angstroms (recall, an angstrom is 10-10 meter or 0.1 nanometer).
However, there are many planes of one direction in any real crystal, even a very small one. X-ray diffraction as a method uses this fact: all waves that have changed direction on planes of the same direction are summed up, giving a fairly clear signal at the output. So scientists can understand in what directions these planes are located inside the crystal, and judge the internal structure of the crystal structure. However, these data alone are not enough. In addition to the angle of inclination, you also need to know the distance between the planes. Without this, you can get thousands of different models of the structure, but not know the exact answer. How scientists learn about the distance between the planes will be discussed below.
Diffraction phenomenon
We have already given a physical justification of what X-ray diffraction on the spatial lattice of crystals is. However, we have not yet explained the essencediffraction phenomena. So, diffraction is the rounding of obstacles by waves (including electromagnetic ones). This phenomenon seems to be a violation of the law of linear optics, but it is not. It is closely related to the interference and wave properties of, for example, photons. If there is an obstacle in the way of light, then due to diffraction, photons can “look” around the corner. How far the direction of light travels from a straight line depends on the size of the obstacle. The smaller the obstacle, the shorter the electromagnetic wave length should be. That is why X-ray diffraction on single crystals is carried out using such short waves: the distance between the planes is very small, optical photons simply will not “crawl” between them, but will only be reflected from the surface.
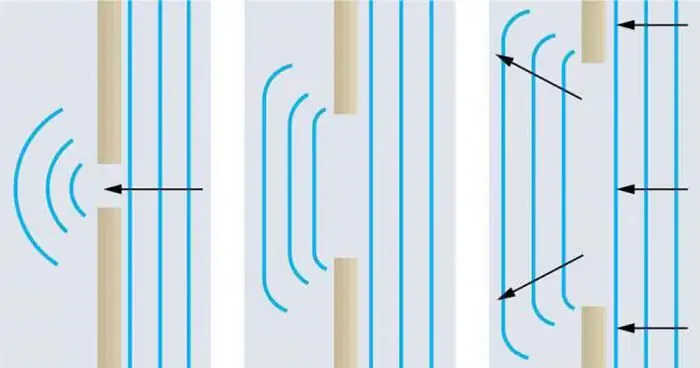
Such a concept is true, but in modern science it is considered too narrow. To expand its definition, as well as for general erudition, we present methods for the manifestation of wave diffraction.
- Changing the spatial structure of waves. For example, the expansion of the propagation angle of a wave beam, the deflection of a wave or a series of waves in some preferred direction. It is to this class of phenomena that wave bending around obstacles belongs.
- Decomposition of waves into a spectrum.
- Change in wave polarization.
- Transformation of the phase structure of waves.
The phenomenon of diffraction, together with interference, is responsible for the fact that when a beam of light is directed to a narrow slit behind it, we see not one, but severallight maxima. The farther the maximum is from the middle of the slot, the higher its order. In addition, with the correct setting of the experiment, the shadow from an ordinary sewing needle (of course, thin) is divided into several stripes, and the light maximum is observed exactly behind the needle, and not the minimum.
Wulf-Bragg formula
We have already said above that the final signal is the sum of all X-ray photons that are reflected from planes with the same inclination inside the crystal. But one important relation allows you to accurately calculate the structure. Without it, X-ray diffraction would be useless. The Wulf-Bragg formula looks like this: 2dsinƟ=nλ. Here d is the distance between planes with the same inclination angle, θ is the glancing angle (Bragg angle), or the angle of incidence on the plane, n is the order of the diffraction maximum, λ is the wavelength. Since it is known in advance which X-ray spectrum is used to obtain data and at what angle this radiation falls, this formula allows us to calculate the value of d. We have already said a little higher that without this information it is impossible to accurately obtain the structure of a substance.
Modern application of X-ray diffraction
The question arises: in what cases is this analysis needed, don't scientists already study everything in the world of structure, and don't people, when obtaining fundamentally new substances, assume what kind of result awaits them? There are four answers.
- Yes, we got to know our planet quite well. But every year new minerals are found. Sometimes their structure is evenguess without x-rays will not work.
- Many scientists are trying to improve the properties of already existing materials. These substances are subjected to various types of processing (pressure, temperature, lasers, etc.). Sometimes elements are added to or removed from their structure. X-ray diffraction on crystals will help to understand what internal rearrangements occurred in this case.
- For some applications (eg, active media, lasers, memory cards, optical elements of surveillance systems), crystals must be very precisely matched. Therefore, their structure is checked using this method.
- X-ray diffraction is the only way to find out how many and which phases were obtained during synthesis in multicomponent systems. Ceramic elements of modern technology can serve as an example of such systems. The presence of unwanted phases can lead to serious consequences.
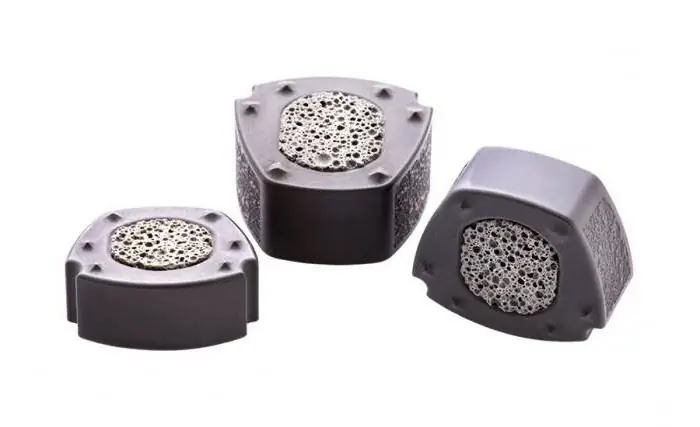
Space exploration
Many people ask: "Why do we need huge observatories in Earth's orbit, why do we need a rover if humanity has not yet solved the problems of poverty and war?"
Everyone has their own reasons for and against, but it is clear that humanity must have a dream.
Therefore, looking at the stars, today we can say with confidence: we know more and more about them every day.
X-rays from processes occurring in space do not reach the surface of our planet, they are absorbed by the atmosphere. But this partThe electromagnetic spectrum carries a lot of data about high-energy phenomena. Therefore, instruments that study X-rays must be taken out of the Earth, into orbit. Currently existing stations are studying the following objects:
- remnants of supernova explosions;
- centers of galaxies;
- neutron stars;
- black holes;
- collisions of massive objects (galaxies, groups of galaxies).
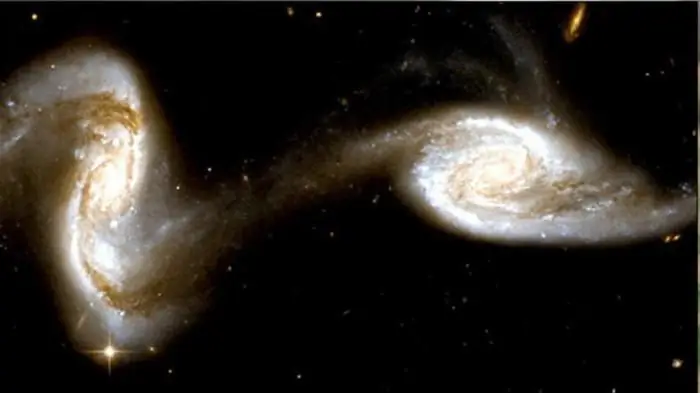
Surprisingly, according to various projects, access to these stations is provided to students and even schoolchildren. They study X-rays coming from deep space: diffraction, interference, spectrum become the subject of their interest. And some very young users of these space observatories are making discoveries. A meticulous reader may, of course, object that they just have time to look at high-resolution pictures and notice subtle details. And of course, the importance of discoveries, as a rule, is understood only by serious astronomers. But such cases inspire young people to dedicate their lives to space exploration. And this goal is worth pursuing.
Thus, the achievements of Wilhelm Conrad Roentgen opened up access to stellar knowledge and the ability to conquer other planets.