Magnetic properties of a material are a class of physical phenomena mediated by fields. Electric currents and magnetic moments of elementary particles generate a field that acts on other currents. The most familiar effects occur in ferromagnetic materials, which are strongly attracted by magnetic fields and can become permanently magnetized, creating the charged fields themselves.
Only a few substances are ferromagnetic. To determine the level of development of this phenomenon in a particular substance, there is a classification of materials according to magnetic properties. The most common are iron, nickel and cob alt and their alloys. The prefix ferro- refers to iron because permanent magnetism was first observed in empty iron, a form of natural iron ore called the magnetic properties of the material, Fe3O4.
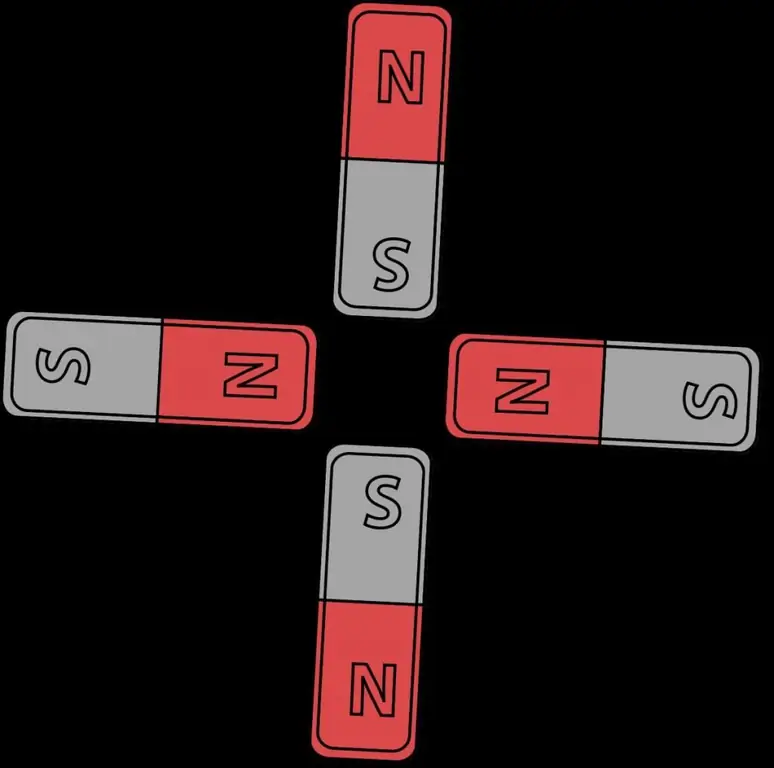
Paramagnetic materials
Althoughferromagnetism is responsible for most of the effects of magnetism encountered in everyday life, all other materials are affected by the field to some extent, as well as some other types of magnetism. Paramagnetic substances such as aluminum and oxygen are weakly attracted to an applied magnetic field. Diamagnetic substances such as copper and carbon repel weakly.
While antiferromagnetic materials such as chromium and spin glasses have a more complex relationship with the magnetic field. The strength of a magnet on paramagnetic, diamagnetic, and antiferromagnetic materials is usually too weak to be felt and can only be detected by laboratory instruments, so these substances are not included in the list of materials that have magnetic properties.
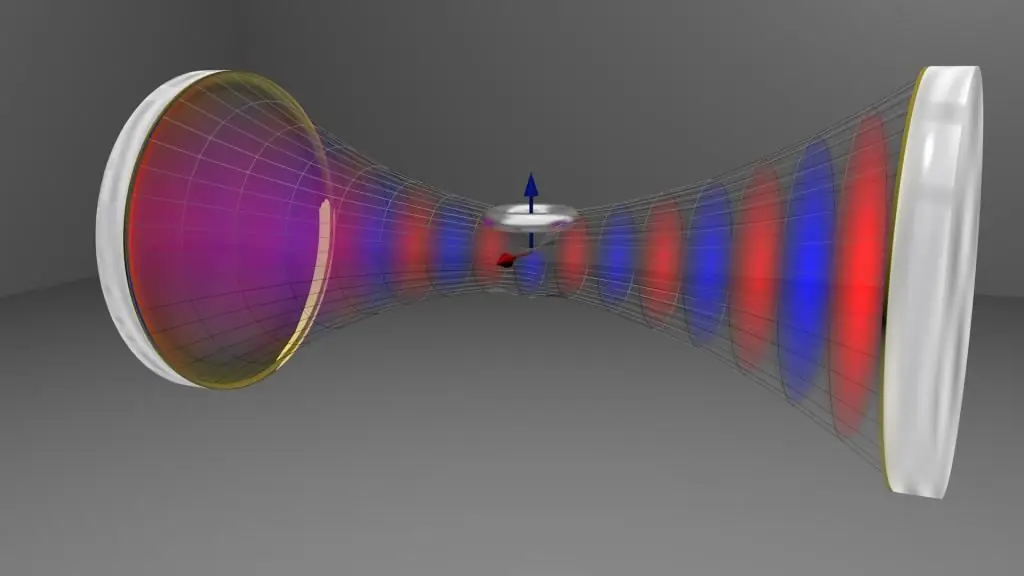
Conditions
The magnetic state (or phase) of a material depends on temperature and other variables such as pressure and applied magnetic field. A material can exhibit more than one form of magnetism as these variables change.
History
The magnetic properties of a material were first discovered in the ancient world when people noticed that magnets, naturally magnetized pieces of minerals, could attract iron. The word "magnet" comes from the Greek term Μαγνῆτις λίθος magnētis lithos, "magnesian stone, footstone".
In ancient Greece, Aristotle attributed the first of what might be called a scientific discussion about the magnetic properties of materials,philosopher Thales of Miletus, who lived from 625 BC. e. before 545 BC e. The ancient Indian medical text Sushruta Samhita describes the use of magnetite to remove arrows embedded in the human body.
Ancient China
In ancient China, the earliest literary reference to the electrical and magnetic properties of materials is found in a 4th-century BC book named after its author, The Sage of the Valley of Ghosts. The earliest mention of needle attraction is in the 1st century work Lunheng (Balanced Requests): "The magnet attracts the needle."
The 11th century Chinese scientist Shen Kuo was the first person to describe - in the Dream Pool Essay - a magnetic compass with a needle and that it improved the accuracy of navigation through astronomical methods. concept of true north. By the 12th century, the Chinese were known to use a magnet compass for navigation. They fashioned the guide spoon out of stone so that the handle of the spoon always points south.
Middle Ages
Alexander Neckam, by 1187, was the first in Europe to describe the compass and its use for navigation. This researcher for the first time in Europe thoroughly established the properties of magnetic materials. In 1269 Peter Peregrine de Maricourt wrote the Epistola de magnete, the first surviving treatise describing the properties of magnets. In 1282, the properties of compasses and materials with special magnetic properties were described by al-Ashraf, a Yemeni physicist, astronomer and geographer.
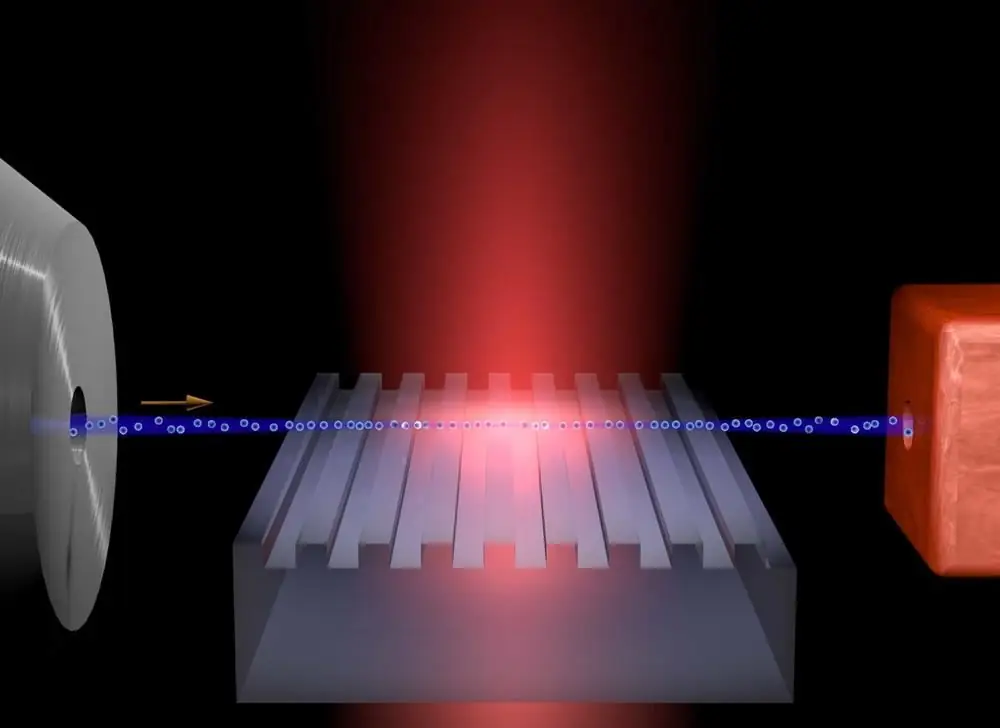
Renaissance
In 1600, William Gilbert publishedhis “Magnetic Corpus” and “Magnetic Tellurium” (“On the Magnet and Magnetic Bodies, and also on the Great Earth Magnet”). In this paper, he describes many of his experiments with his model earth, called the terrella, with which he conducted research on the properties of magnetic materials.
From his experiments, he came to the conclusion that the Earth itself is magnetic and that this is why compasses pointed north (earlier, some believed that it was the pole star (Polaris) or a large magnetic island at the North Pole that attracted the compass).
New time
Understanding of the relationship between electricity and materials with special magnetic properties appeared in 1819 in the work of Hans Christian Oersted, a professor at the University of Copenhagen, who discovered by accidentally jerking a compass needle near a wire that an electric current could create a magnetic field. This landmark experiment is known as the Oersted Experiment. Several other experiments followed with André-Marie Ampère, who in 1820 discovered that a magnetic field circulating in a closed path was related to a current flowing around the perimeter of the path.
Carl Friedrich Gauss was engaged in the study of magnetism. Jean-Baptiste Biot and Felix Savart in 1820 came up with the Biot-Savart law, which gives the desired equation. Michael Faraday, who discovered in 1831 that a time-varying magnetic flux through a loop of wire caused a voltage. And other scientists have found further connections between magnetism and electricity.
XX century and ourtime
James Clerk Maxwell synthesized and extended this understanding of Maxwell's equations by unifying electricity, magnetism and optics in the field of electromagnetism. In 1905, Einstein used these laws to motivate his theory of special relativity by requiring that the laws hold true in all inertial reference frames.
Electromagnetism has continued to evolve into the 21st century, being incorporated into the more fundamental theories of gauge theory, quantum electrodynamics, the electroweak theory, and finally the standard model. Nowadays, scientists are already studying the magnetic properties of nanostructured materials with might and main. But the greatest and most amazing discoveries in this field are probably still ahead of us.
Essence
The magnetic properties of materials are mainly due to the magnetic moments of the orbital electrons of their atoms. The magnetic moments of atomic nuclei are usually thousands of times smaller than those of electrons, and therefore they are negligible in the context of the magnetization of materials. Nuclear magnetic moments are nevertheless very important in other contexts, especially in nuclear magnetic resonance (NMR) and magnetic resonance imaging (MRI).
Usually, the huge number of electrons in a material is arranged in such a way that their magnetic moments (both orbital and internal) are nullified. To some extent, this is due to the fact that electrons combine in pairs with opposite intrinsic magnetic moments as a result of the Pauli principle (see Electron configuration) and combine into filled subshells with zero net orbital motion.
BIn both cases, the electrons predominantly use circuits in which the magnetic moment of each electron is canceled out by the opposite moment of the other electron. Moreover, even when the electron configuration is such that there are unpaired electrons and/or unfilled subshells, it is often the case that different electrons in a solid will contribute magnetic moments that point in different, random directions, so that the material will not be magnetic.
Sometimes, either spontaneously or due to an applied external magnetic field, each of the electrons' magnetic moments will line up on average. The right material can then create a strong net magnetic field.
The magnetic behavior of a material depends on its structure, in particular on its electronic configuration, for the reasons given above, and also on temperature. At high temperatures, random thermal motion makes it difficult for electrons to align.
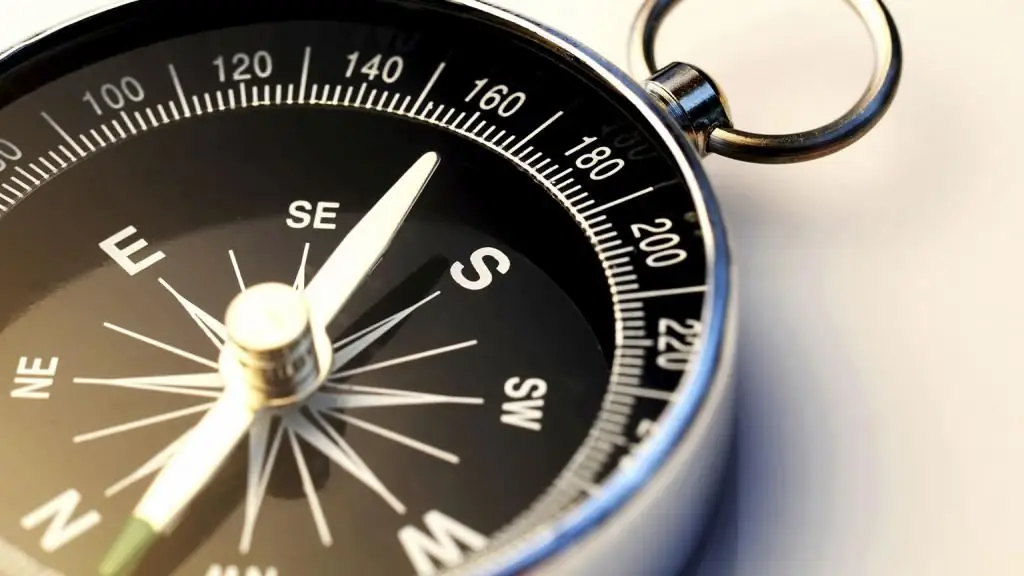
Diamagnetism
Diamagnetism is found in all materials and is the tendency of a material to resist an applied magnetic field and therefore repel the magnetic field. However, in a material with paramagnetic properties (that is, with a tendency to strengthen an external magnetic field), the paramagnetic behavior dominates. Thus, despite the universal occurrence, diamagnetic behavior is observed only in a purely diamagnetic material. There are no unpaired electrons in a diamagnetic material, so the intrinsic magnetic moments of electrons cannot createany volume effect.
Please note that this description is intended as a heuristic only. The Bohr-Van Leeuwen theorem shows that diamagnetism is impossible according to classical physics, and that a correct understanding requires a quantum mechanical description.
Note that all materials go through this orbital response. However, in paramagnetic and ferromagnetic substances, the diamagnetic effect is suppressed by much stronger effects caused by unpaired electrons.
There are unpaired electrons in a paramagnetic material; that is, atomic or molecular orbitals with exactly one electron in them. While the Pauli exclusion principle requires paired electrons to have their own ("spin") magnetic moments pointing in opposite directions, causing their magnetic fields to cancel out, an unpaired electron can align its magnetic moment in either direction. When an external field is applied, these moments will tend to align in the same direction as the applied field, strengthening it.
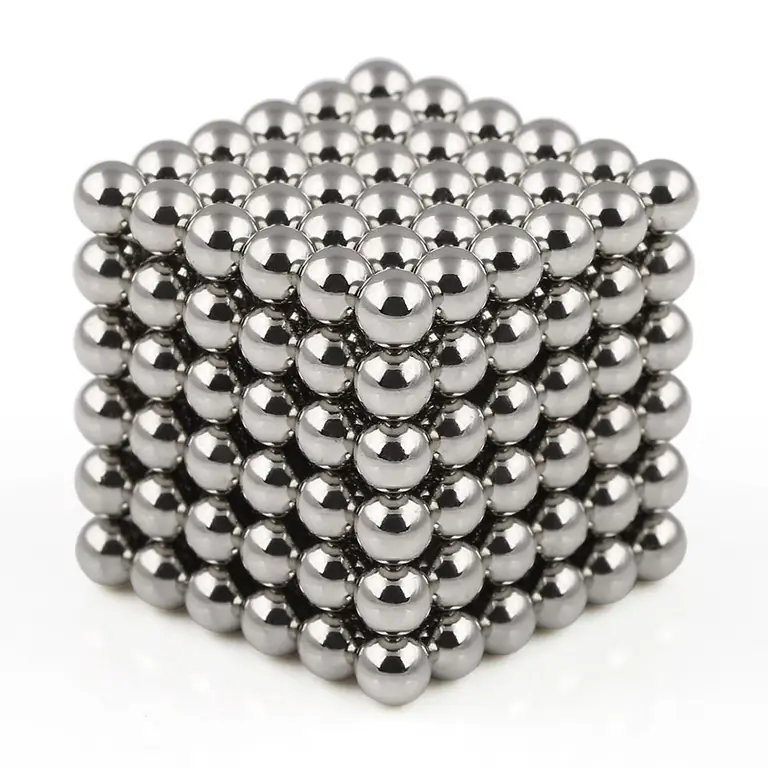
Ferromagnets
A ferromagnet, as a paramagnetic substance, has unpaired electrons. However, in addition to the tendency of the intrinsic magnetic moment of the electrons to be parallel to the applied field, in these materials there is also a tendency for these magnetic moments to orient themselves parallel to each other in order to maintain a state of reduced energy. Thus, even in the absence of an applied fieldthe magnetic moments of the electrons in the material spontaneously line up parallel to each other.
Each ferromagnetic substance has its own individual temperature, called the Curie temperature, or the Curie point, above which it loses its ferromagnetic properties. This is because the thermal tendency to disorder overwhelms the reduction in energy due to ferromagnetic order.
Ferromagnetism only occurs in a few substances; iron, nickel, cob alt, their alloys, and some rare earth alloys are common.
The magnetic moments of atoms in a ferromagnetic material cause them to behave like tiny permanent magnets. They stick together and combine into small regions of more or less uniform alignment called magnetic domains or Weiss domains. Magnetic domains can be observed using a magnetic force microscope to reveal magnetic domain boundaries that resemble white lines in a sketch. There are many scientific experiments that can physically show magnetic fields.
Role of domains
When a domain contains too many molecules, it becomes unstable and splits into two domains aligned in opposite directions to stick together more stably, as shown on the right.
When exposed to a magnetic field, the domain boundaries move so that magnetically aligned domains grow and dominate the structure (dotted yellow area), as shown on the left. When the magnetizing field is removed, the domains may not return to an unmagnetized state. This leads tobecause the ferromagnetic material is magnetized, forming a permanent magnet.
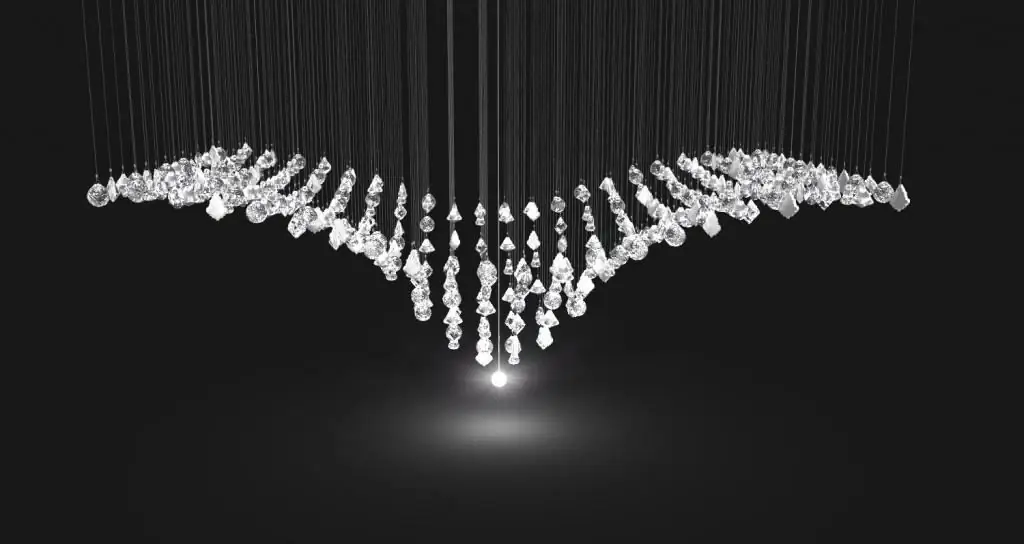
When the magnetization was strong enough so that the dominant domain overlapped all the others, leading to the formation of only one separate domain, the material was magnetically saturated. When a magnetized ferromagnetic material is heated to the Curie point temperature, the molecules mix to the point where the magnetic domains lose organization and the magnetic properties they cause cease. When the material is cooled, this domain alignment structure spontaneously returns, roughly analogous to how a liquid can freeze into a crystalline solid.
Antiferromagnetics
In an antiferromagnet, unlike a ferromagnet, the intrinsic magnetic moments of neighboring valence electrons tend to point in opposite directions. When all the atoms are arranged in a substance so that each neighbor is antiparallel, the substance is antiferromagnetic. Antiferromagnets have a net magnetic moment of zero, which means they do not create a field.
Antiferromagnets are rarer than other types of behavior and are most often observed at low temperatures. At different temperatures, antiferromagnets exhibit diamagnetic and ferromagnetic properties.
In some materials, neighboring electrons prefer to point in opposite directions, but there is no geometric arrangement in which each pair of neighbors is anti-aligned. It's called spin glass andis an example of geometric frustration.
Magnetic properties of ferromagnetic materials
Like ferromagnetism, ferrimagnets retain their magnetization in the absence of a field. However, like antiferromagnets, adjacent pairs of electron spins tend to point in opposite directions. These two properties do not contradict each other because, in an optimal geometric arrangement, the magnetic moment from a sublattice of electrons that point in the same direction is greater than from a sublattice that points in the opposite direction.
Most ferrites are ferrimagnetic. The magnetic properties of ferromagnetic materials today are considered undeniable. The first discovered magnetic substance, magnetite, is a ferrite and was originally thought to be a ferromagnet. However, Louis Neel disproved this by discovering ferrimagnetism.
When a ferromagnet or ferrimagnet is small enough, it acts as a single magnetic spin that is subject to Brownian motion. Its response to a magnetic field is qualitatively similar to that of a paramagnet, but much more.
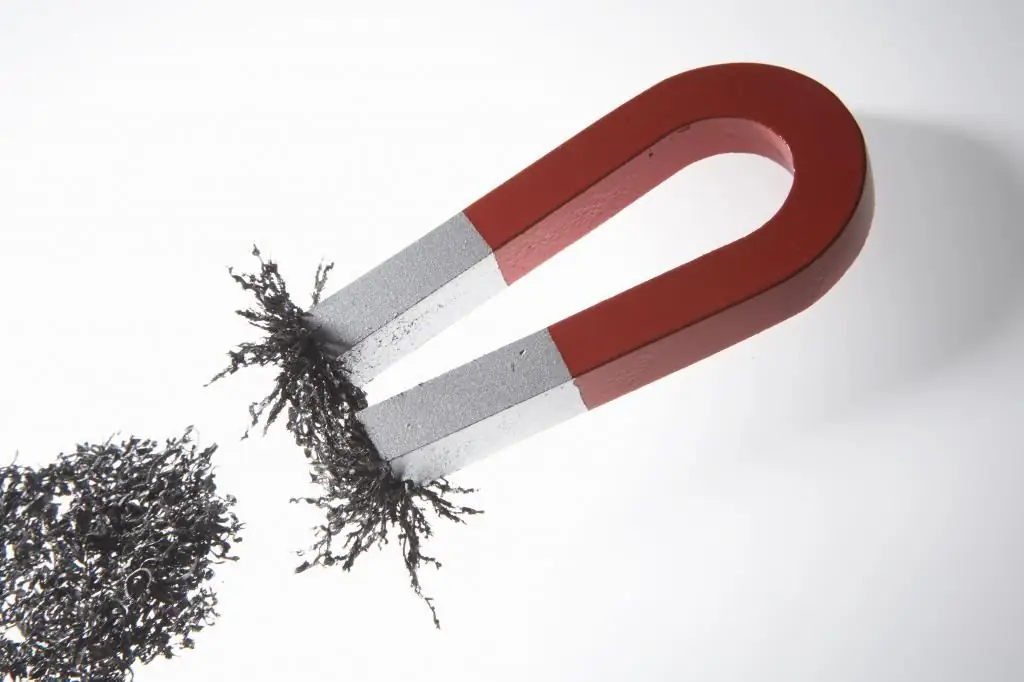
Electromagnets
An electromagnet is a magnet in which a magnetic field is created by an electric current. The magnetic field disappears when the current is turned off. Electromagnets usually consist of a large number of closely spaced turns of wire that create a magnetic field. Wire coils are often wound around a magnetic core made of ferromagnetic or ferrimagnetic material.a material such as iron; the magnetic core concentrates the magnetic flux and creates a stronger magnet.
The main advantage of an electromagnet over a permanent magnet is that the magnetic field can be quickly changed by controlling the amount of electric current in the winding. However, unlike a permanent magnet, which does not require power, an electromagnet requires a continuous supply of current to maintain the magnetic field.
Electromagnets are widely used as components of other electrical devices such as motors, generators, relays, solenoids, loudspeakers, hard drives, MRI machines, scientific instruments and magnetic separation equipment. Electromagnets are also used in industry to pick up and move heavy iron objects such as scrap metal and steel. Electromagnetism was discovered in 1820. At the same time, the first classification of materials according to magnetic properties was published.