A neutrino is an elementary particle that is very similar to an electron, but has no electric charge. It has a very small mass, which may even be zero. The speed of the neutrino also depends on the mass. The difference in the arrival time of the particle and light is 0.0006% (± 0.0012%). In 2011, during the OPERA experiment, it was found that the speed of neutrinos exceeds the speed of light, but independent experience did not confirm this.
The Elusive Particle
This is one of the most common particles in the universe. Since it interacts very little with matter, it is incredibly difficult to detect. Electrons and neutrinos do not participate in strong nuclear interactions, but equally participate in weak ones. Particles with these properties are called leptons. In addition to the electron (and its antiparticle, the positron), charged leptons include the muon (200 electron masses), the tau (3500 electron masses), and their antiparticles. They are called so: electron-, muon- and tau-neutrinos. They each have an anti-material component called an antineutrino.
Muon and tau, like an electron, have particles accompanying them. These are muon and tau neutrinos. The three types of particles are different from each other. For example, when muon neutrinos interact with a target, they always produce muons, never tau or electrons. In the interaction of particles, although electrons and electron-neutrinos can be created and destroyed, their sum remains unchanged. This fact leads to the division of leptons into three types, each of which has a charged lepton and an accompanying neutrino.
Very large and extremely sensitive detectors are needed to detect this particle. Typically, low-energy neutrinos will travel many light-years before interacting with matter. Consequently, all ground-based experiments with them rely on measuring their small fraction interacting with recorders of reasonable size. For example, at the Sudbury Neutrino Observatory, containing 1000 tons of heavy water, about 1012 solar neutrinos per second pass through the detector. And only 30 a day are found.
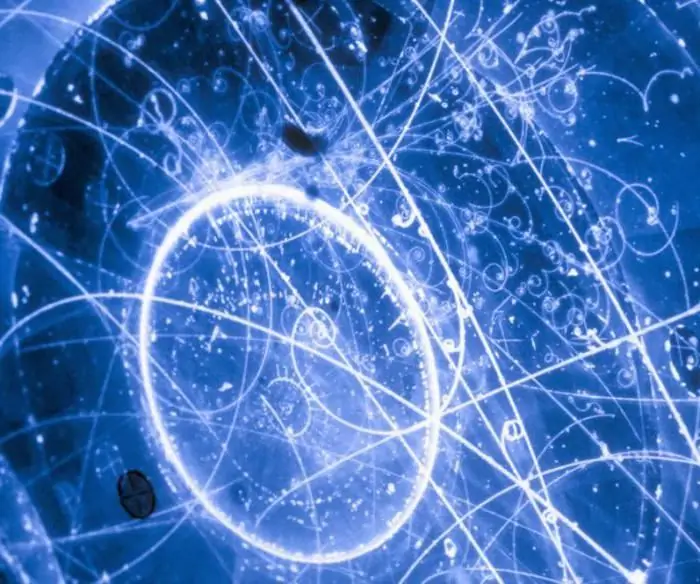
Discovery history
Wolfgang Pauli first postulated the existence of a particle in 1930. A problem arose at the time because it seemed that energy and angular momentum were not conserved in beta decay. But Pauli noted that if a non-interacting neutral neutrino particle is emitted, then the law of conservation of energy will be observed. The Italian physicist Enrico Fermi developed the theory of beta decay in 1934 and gave the particle its name.
Despite all the predictions, for 20 years neutrinos could not be detected experimentally because of its weak interaction with matter. Since the particles are not electricallycharged, they are not affected by electromagnetic forces, and, therefore, they do not cause ionization of matter. In addition, they react with matter only through weak interactions of negligible strength. Therefore, they are the most penetrating subatomic particles, able to pass through a huge number of atoms without causing any reaction. Only 1 in 10 billion of these particles, traveling through matter a distance equal to the diameter of the Earth, reacts with a proton or neutron.
Finally, in 1956, a group of American physicists led by Frederick Reines announced the discovery of the electron-antineutrino. In her experiments, antineutrinos emitted from a nuclear reactor interacted with protons to form neutrons and positrons. The unique (and rare) energy signatures of these latest by-products provide evidence for the existence of the particle.
The discovery of charged muon leptons became the starting point for the subsequent identification of the second type of neutrino - muon. Their identification was carried out in 1962 based on the results of an experiment in a particle accelerator. High-energy muonic neutrinos were produced by the decay of pi-mesons and sent to the detector in such a way that their reactions with matter could be studied. Although they are non-reactive, like other types of these particles, it has been found that on the rare occasions when they react with protons or neutrons, muon-neutrinos form muons, but never electrons. In 1998, American physicists Leon Lederman, Melvin Schwartz and Jack Steinbergerreceived the Nobel Prize in Physics for the identification of the muon-neutrino.
In the mid-1970s, neutrino physics was replenished with another type of charged leptons - tau. The tau neutrino and tau antineutrino turned out to be associated with this third charged lepton. In 2000, physicists at the National Accelerator Laboratory. Enrico Fermi reported the first experimental evidence for the existence of this type of particle.
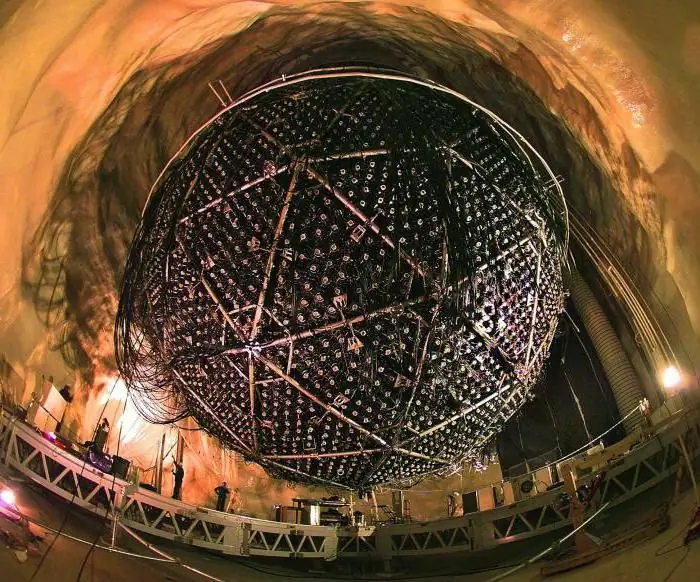
Mass
All types of neutrinos have a mass that is much less than that of their charged counterparts. For example, experiments show that the electron-neutrino mass must be less than 0.002% of the electron mass and that the sum of the masses of the three species must be less than 0.48 eV. For many years it seemed that the mass of a particle was zero, although there was no convincing theoretical evidence why this should be so. Then, in 2002, the Sudbury Neutrino Observatory provided the first direct evidence that electron-neutrinos emitted by nuclear reactions in the Sun's core change type as they travel through it. Such "oscillations" of neutrinos are possible if one or more types of particles have some small mass. Their studies of the interaction of cosmic rays in the Earth's atmosphere also indicate the presence of mass, but further experiments are required to determine it more accurately.
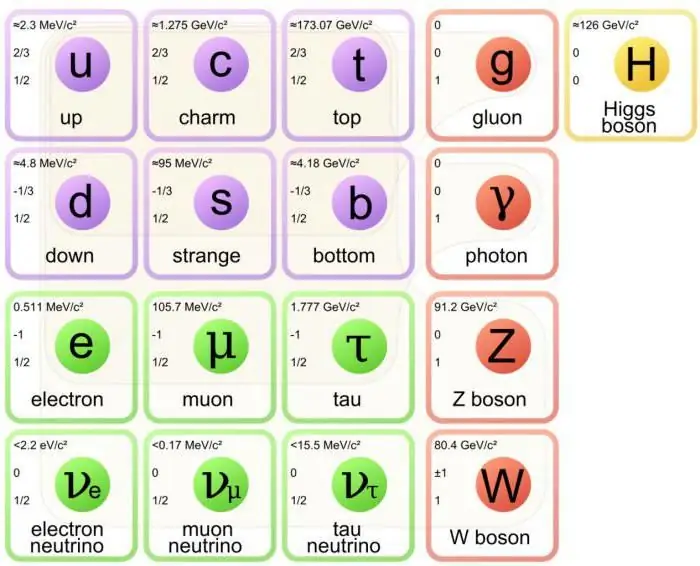
Sources
Natural sources of neutrinos are the radioactive decay of elements in the bowels of the Earth, in whicha large stream of low-energy electrons-antineutrinos is emitted. Supernovae are also a predominantly neutrino phenomenon, since only these particles can penetrate the superdense material produced in a collapsing star; only a small part of the energy is converted into light. Calculations show that about 2% of the Sun's energy is the energy of neutrinos produced in thermonuclear fusion reactions. It is likely that most of the dark matter in the universe is made up of neutrinos produced during the Big Bang.
Problems of physics
The fields related to neutrinos and astrophysics are diverse and rapidly developing. The current questions attracting a large number of experimental and theoretical efforts are as follows:
- What are the masses of different neutrinos?
- How do they affect Big Bang cosmology?
- Do they oscillate?
- Can neutrinos of one type transform into another as they travel through matter and space?
- Are neutrinos fundamentally different from their antiparticles?
- How do stars collapse and form supernovae?
- What is the role of neutrinos in cosmology?
One of the longstanding problems of particular interest is the so-called solar neutrino problem. This name refers to the fact that during several ground-based experiments conducted over the past 30 years, fewer particles were consistently observed than needed to produce energy emitted by the sun. One of its possible solutions is oscillation, i.e., the transformation of electronicneutrinos into muons or tau while traveling to Earth. Since it is much more difficult to measure low-energy muon or tau neutrinos, this kind of transformation could explain why we do not observe the correct number of particles on Earth.
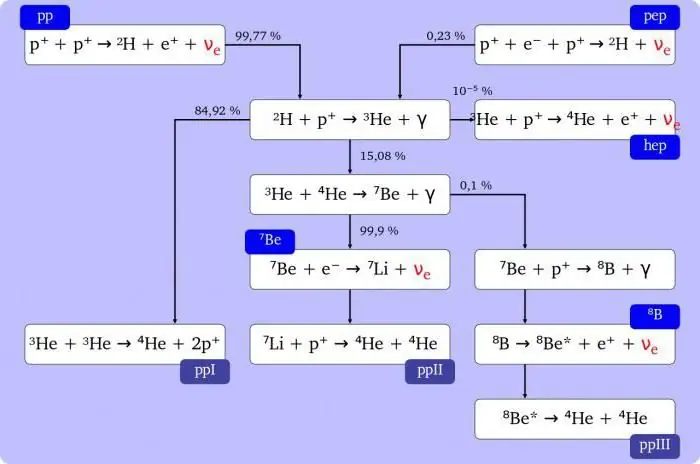
Fourth Nobel Prize
The 2015 Nobel Prize in Physics was awarded to Takaaki Kajita and Arthur McDonald for their discovery of the neutrino mass. This was the fourth such award related to experimental measurements of these particles. Some might wonder why we should care so much about something that barely interacts with ordinary matter.
The very fact that we can detect these ephemeral particles is a testament to human ingenuity. Since the rules of quantum mechanics are probabilistic, we know that even though almost all neutrinos pass through the Earth, some of them will interact with it. A detector large enough to detect this.
The first such device was built in the sixties deep in a mine in South Dakota. The mine was filled with 400 thousand liters of cleaning fluid. On average, one neutrino particle every day interacts with a chlorine atom, turning it into argon. Incredibly, Raymond Davis, who was in charge of the detector, came up with a way to detect these few argon atoms, and four decades later, in 2002, he was awarded the Nobel Prize for this amazing technical feat.
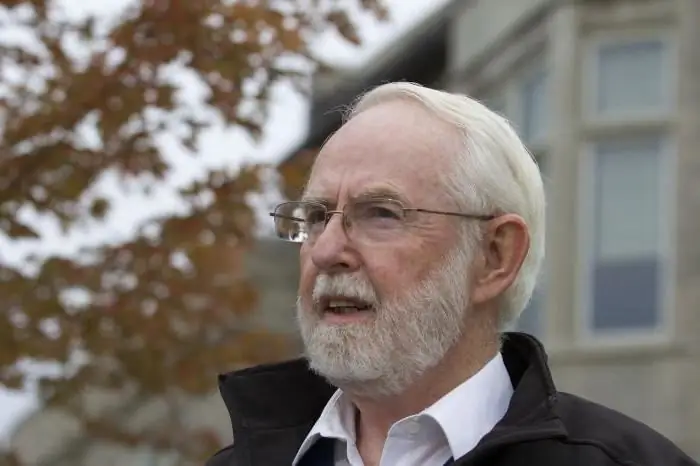
New Astronomy
Because neutrinos interact so weakly, they can travel great distances. They give us the opportunity to look into places that we would otherwise never see. The neutrinos Davis discovered were produced by nuclear reactions that took place in the very center of the Sun, and were able to escape this incredibly dense and hot place only because they hardly interact with other matter. It is even possible to detect a neutrino flying from the center of an exploding star over a hundred thousand light-years from Earth.
In addition, these particles make it possible to observe the universe on a very small scale, much smaller than what the Large Hadron Collider in Geneva, which discovered the Higgs boson, can look into. It is for this reason that the Nobel Committee decided to award the Nobel Prize for the discovery of yet another type of neutrino.
Mysterious Missing
When Ray Davis observed solar neutrinos, he found only a third of the expected number. Most physicists believed that the reason for this was a poor knowledge of the astrophysics of the Sun: perhaps models of the interior of the star overestimated the number of neutrinos produced in it. Yet over the years, even as solar models improved, shortages persisted. Physicists drew attention to another possibility: the problem could be related to our understanding of these particles. According to the then prevailing theory, they had no mass. But some physicists have argued that the particles actually had an infinitesimalmass, and this mass was the reason for their shortage.
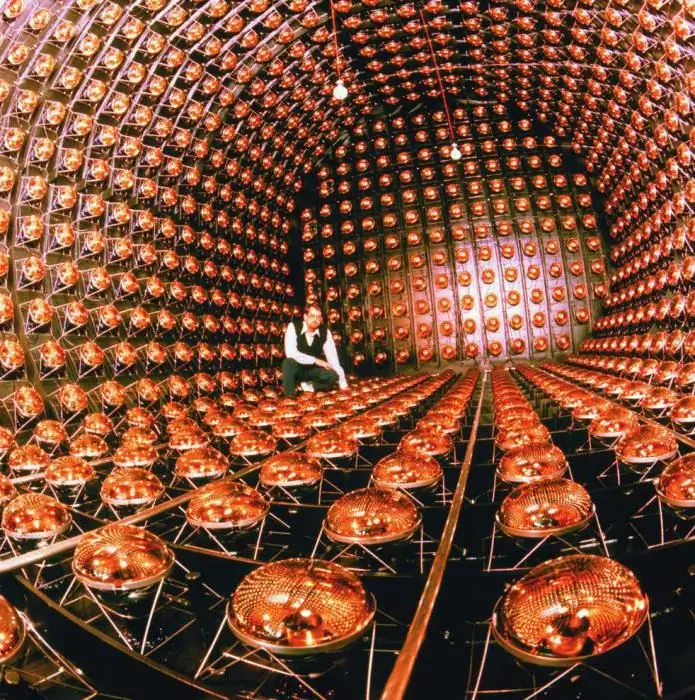
Three-faced particle
According to the theory of neutrino oscillations, there are three different types of neutrinos in nature. If a particle has mass, then as it moves, it can change from one type to another. Three types - electron, muon and tau - when interacting with matter can be converted into the corresponding charged particle (electron, muon or tau lepton). "Oscillation" occurs due to quantum mechanics. The type of neutrino is not constant. It changes over time. A neutrino, which began its existence as an electron, can turn into a muon, and then back. Thus, a particle formed in the core of the Sun, on its way to the Earth, can periodically turn into a muon-neutrino and vice versa. Since the Davis detector could only detect electron neutrinos capable of leading to the nuclear transmutation of chlorine into argon, it seemed possible that the missing neutrinos had turned into other types. (As it turns out, neutrinos oscillate inside the Sun, not on their way to Earth.)
Canadian experiment
The only way to test this was to build a detector that worked for all three types of neutrinos. Since the 1990s, Arthur McDonald of Queen's Ontario University has led the team that did this in a mine in Sudbury, Ontario. The facility contained tons of heavy water on loan from the Canadian government. Heavy water is a rare but naturally occurring form of water in which hydrogen, containing one proton,replaced by its heavier isotope deuterium, which contains a proton and a neutron. The Canadian government stockpiled heavy water because it is used as a coolant in nuclear reactors. All three types of neutrinos could destroy deuterium to form a proton and a neutron, and the neutrons were then counted. The detector registered about three times the number of particles compared to Davis - exactly the number that was predicted by the best models of the Sun. This suggested that the electron-neutrino could oscillate into its other types.
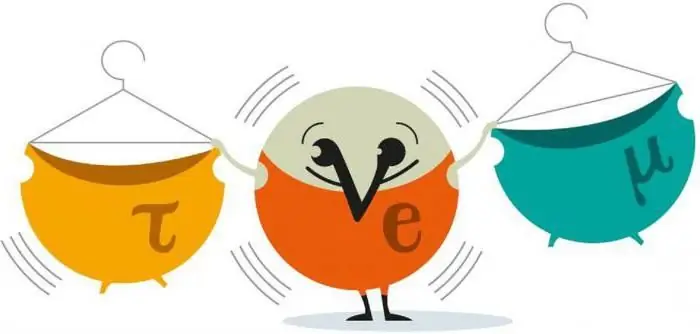
Japanese experiment
Around the same time, Takaaki Kajita of the University of Tokyo was doing another remarkable experiment. A detector installed in a mine in Japan registered neutrinos coming not from the bowels of the Sun, but from the upper atmosphere. When cosmic ray protons collide with the atmosphere, showers of other particles are formed, including muon neutrinos. In the mine, they turned hydrogen nuclei into muons. The Kajita detector could see particles coming in two directions. Some fell from above, coming from the atmosphere, while others moved from below. The number of particles was different, which indicated their different nature - they were at different points of their oscillation cycles.
Revolution in science
It's all exotic and amazing, but why do oscillations and neutrino masses attract so much attention? The reason is simple. In the standard model of particle physics developed over the last fifty years of the twentieth century,which correctly described all other observations in accelerators and other experiments, neutrinos should have been massless. The discovery of the neutrino mass suggests that something is missing. The Standard Model is not complete. The missing elements have yet to be discovered, either through the Large Hadron Collider or another yet-to-be-created machine.