In simple terms, the Higgs boson is the most expensive particle of all time. If, for example, a vacuum tube and a couple of brilliant minds were enough to discover the electron, the search for the Higgs boson required the creation of experimental energy, which is rarely found on Earth. The Large Hadron Collider needs no introduction, being one of the most famous and successful scientific experiments, but its profile particle, as before, is shrouded in mystery for most of the population. It has been called a God particle, however, thanks to the efforts of literally thousands of scientists, we no longer have to accept its existence on faith.
Last unknown
What is the Higgs boson and what is the importance of its discovery? Why has it become the subject of so much hype, funding and misinformation? For two reasons. First, it was the last undiscovered particle needed to confirm the Standard Model of physics. Her discovery meant that a whole generation of scientific publications had not been in vain. Secondly, this boson gives other particles their mass, which gives it a special meaning and some "magic". We tend to think aboutmass as an intrinsic property of things, but physicists think otherwise. In simple terms, the Higgs boson is a particle without which mass does not exist in principle.
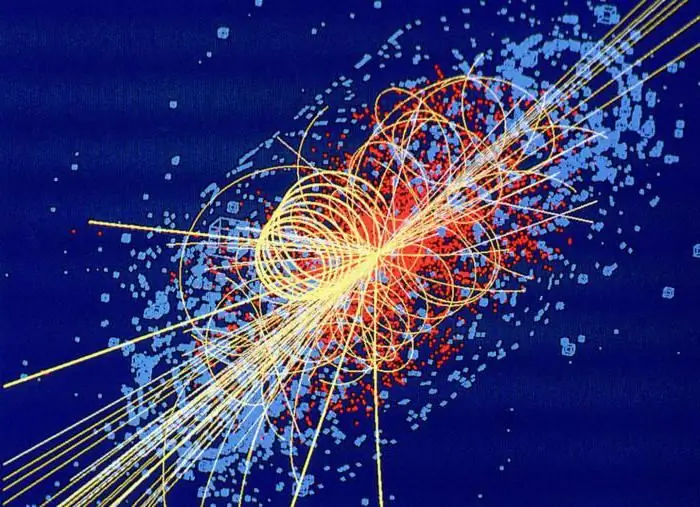
One more field
The reason lies in the so-called Higgs field. It was described even before the Higgs boson, because physicists calculated it for the needs of their own theories and observations, which required the presence of a new field, the action of which would extend to the entire Universe. Reinforcing hypotheses by inventing new components of the universe is dangerous. In the past, for example, this led to the creation of the aether theory. But the more mathematical calculations were made, the more physicists understood that the Higgs field must exist in reality. The only problem was the lack of practical means of observing him.
In the Standard Model of physics, elementary particles gain mass through a mechanism based on the existence of the Higgs field that permeates all of space. It creates Higgs bosons, which require a lot of energy, and this is the main reason why scientists need modern particle accelerators to conduct high-energy experiments.
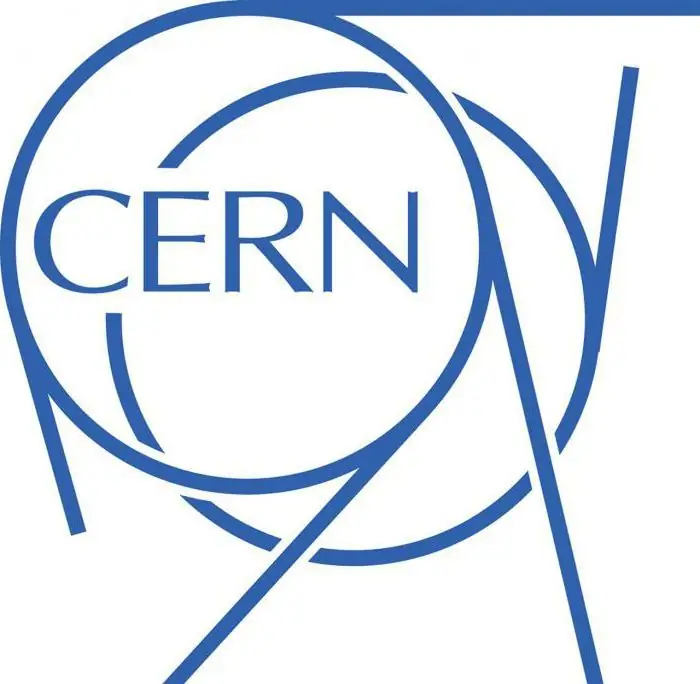
Where does mass come from?
The strength of weak nuclear interactions rapidly decreases with increasing distance. According to quantum field theory, this means that the particles that are involved in its creation - W- and Z-bosons - must have mass, unlike gluons and photons, which have no mass.
The problem is that gauge theories only deal with massless elements. If the gauge bosons have mass, then such a hypothesis cannot be reasonably defined. The Higgs mechanism avoids this problem by introducing a new field called the Higgs field. At high energies, gauge bosons have no mass, and the hypothesis works as expected. At low energies, the field causes a symmetry breaking that allows elements to have mass.
What is the Higgs boson?
The Higgs field produces particles called Higgs bosons. Their mass is not specified by theory, but as a result of the experiment, it was determined that it is equal to 125 GeV. In simple terms, the Higgs boson has definitively confirmed the Standard Model with its existence.
Mechanism, field and boson bear the name of the Scottish scientist Peter Higgs. Although he was not the first to propose these concepts, but, as is often the case in physics, he simply happened to be the one after whom they were named.
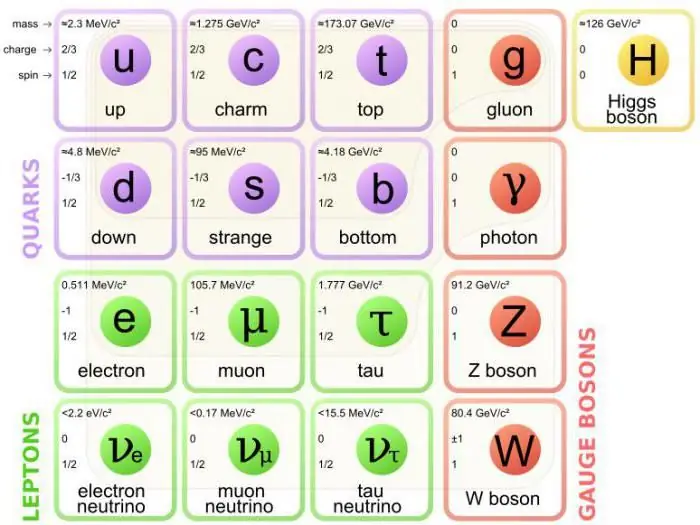
Broken symmetry
The Higgs field was thought to be responsible for the fact that particles that shouldn't have mass did. This is a universal medium that endows massless particles with different masses. Such a violation of symmetry is explained by analogy with light - all wavelengths move in vacuum with the same speed, while in a prism each wavelength can be distinguished. This is, of course, an incorrect analogy, since white light contains all wavelengths, but the example shows howthe creation of mass by the Higgs field appears to be due to symmetry breaking. A prism breaks the symmetry of the speed of different wavelengths of light by separating them, and the Higgs field is thought to break the symmetry of the masses of some particles that are otherwise symmetrically massless.
How to explain the Higgs boson in simple terms? Only recently have physicists realized that if the Higgs field really exists, its operation will require the presence of an appropriate carrier with properties due to which it can be observed. It was assumed that this particle belonged to bosons. In simple terms, the Higgs boson is the so-called carrier force, the same as photons, which are carriers of the electromagnetic field of the Universe. Photons, in a sense, are its local excitations, just as the Higgs boson is a local excitation of its field. Proving the existence of a particle with the properties expected by physicists was, in fact, tantamount to directly proving the existence of a field.
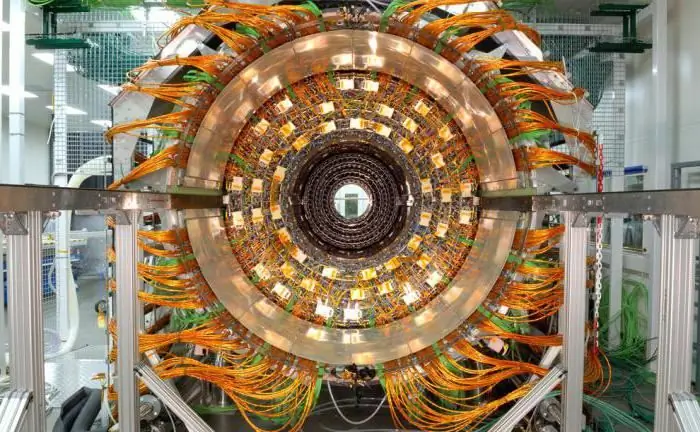
Experiment
Many years of planning have allowed the Large Hadron Collider (LHC) to become a testament to a potential disproof of the Higgs boson theory. A 27-km ring of super-powerful electromagnets can accelerate charged particles to significant fractions of the speed of light, causing collisions strong enough to separate them into their components, as well as deform space around the point of impact. According to calculations, at a collision energy of a sufficiently high level, it is possible to charge a boson so that it decays, and this can bewill watch. This energy was so great that some even panicked and predicted the end of the world, and the fantasy of others went so far that the discovery of the Higgs boson was described as an opportunity to look into an alternative dimension.
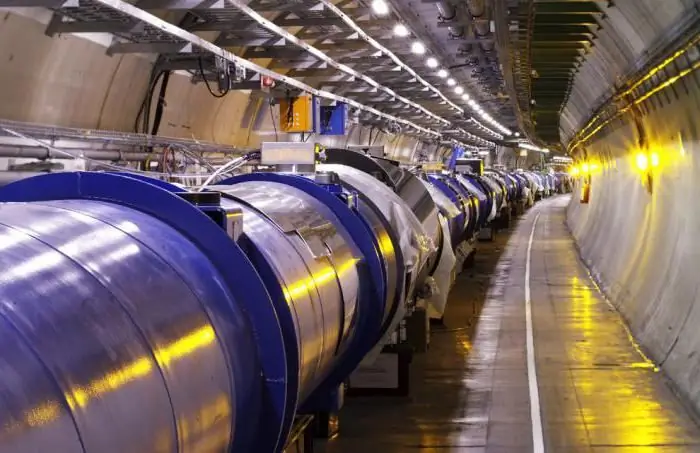
Final confirmation
Initial observations seemed to actually disprove the predictions, and no sign of the particle could be found. Some of the researchers involved in the campaign to spend billions of dollars even appeared on television and meekly stated the fact that refuting a scientific theory is just as important as confirming it. After some time, however, the measurements began to add up to the big picture, and on March 14, 2013, CERN officially announced the confirmation of the particle's existence. There is evidence to suggest the existence of multiple bosons, but this idea needs further study.
Two years after CERN announced the discovery of the particle, scientists working at the Large Hadron Collider were able to confirm it. On the one hand, this was a huge victory for science, and on the other hand, many scientists were disappointed. If anyone had hoped that the Higgs boson would be the particle that would lead to strange and wonderful regions beyond the Standard Model - supersymmetry, dark matter, dark energy - then, unfortunately, this turned out not to be the case.
A study published in Nature Physics has confirmed the decay into fermions. The Standard Model predicts that, in simple terms, the bosonThe Higgs is the particle that gives fermions their mass. The detector of the CMS collider finally confirmed their decay into fermions - down quarks and tau leptons.
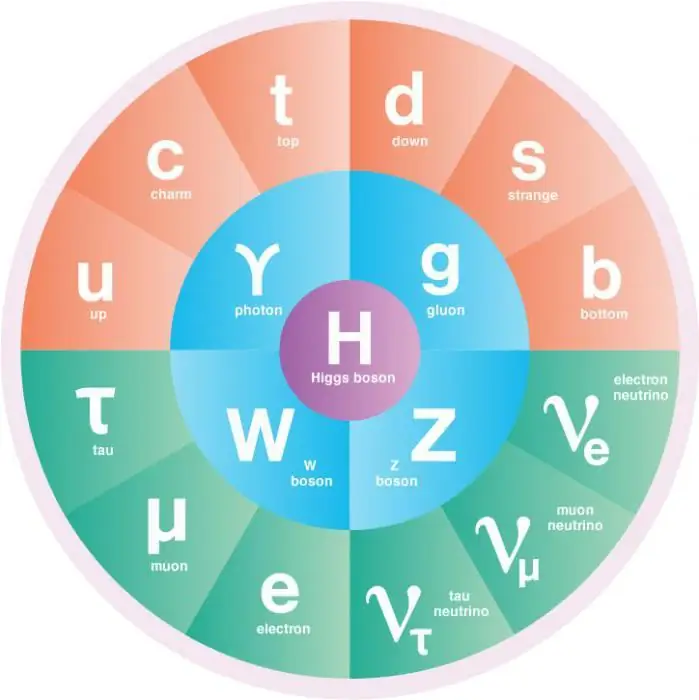
Higgs boson in simple terms: what is it?
This study has finally confirmed that this is the Higgs boson predicted by the Standard Model of particle physics. It is located in the mass-energy region of 125 GeV, has no spin, and can decay into many lighter elements - pairs of photons, fermions, etc. Thanks to this, we can confidently say that the Higgs boson, in simple terms, is a particle giving mass to everything.
Disappointed with the default behavior of a newly opened element. If its decay were even slightly different, it would be related to fermions differently, and new lines of research would emerge. On the other hand, this means that we have not moved a single step beyond the Standard Model, which does not take into account gravity, dark energy, dark matter and other bizarre phenomena of reality.
Now one can only guess what caused them. The most popular theory is supersymmetry, which states that every particle in the Standard Model has an incredibly heavy superpartner (thus making up 23% of the universe - dark matter). Upgrading the collider, doubling its collision energy to 13 TeV, will likely make it possible to detect these superparticles. Otherwise, supersymmetry will have to wait for the construction of a more powerful successor to the LHC.
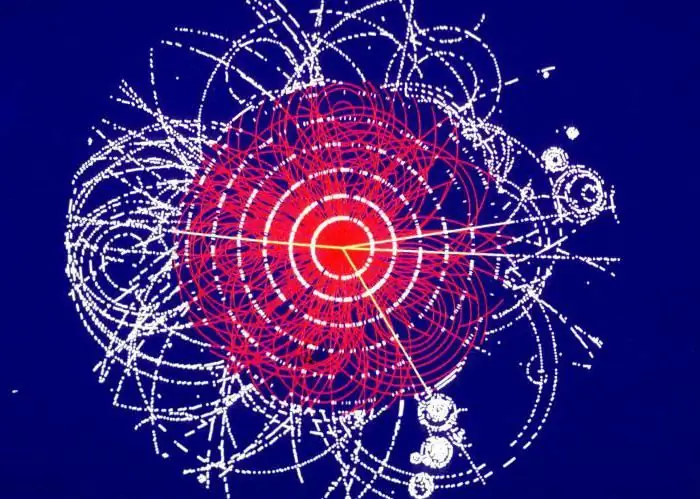
Further prospects
So what will physics be like after the Higgs boson? The LHC has recently resumed its work with significant improvements and is able to see everything from antimatter to dark energy. It is believed that dark matter interacts with ordinary matter solely through gravity and through the creation of mass, and the significance of the Higgs boson is key to understanding exactly how this happens. The main drawback of the Standard Model is that it cannot explain the effects of gravity - such a model could be called the Grand Unified Theory - and some believe that the particle and the Higgs field could be the bridge that physicists are so desperate to find.
The existence of the Higgs boson has been confirmed, but its full understanding is still very far away. Will future experiments refute supersymmetry and the idea of its decomposition into dark matter itself? Or will they confirm every last detail of the Standard Model's predictions about the properties of the Higgs boson and end this area of research forever?