Atomic emission spectroscopy (AES) is a chemical analysis method that uses the intensity of light emitted by a flame, plasma, arc or spark at a specific wavelength to determine the amount of an element in a sample.
The wavelength of an atomic spectral line gives the element's identity, while the intensity of the emitted light is proportional to the number of atoms of the element. This is the essence of atomic emission spectroscopy. It allows you to analyze elements and physical phenomena with impeccable accuracy.
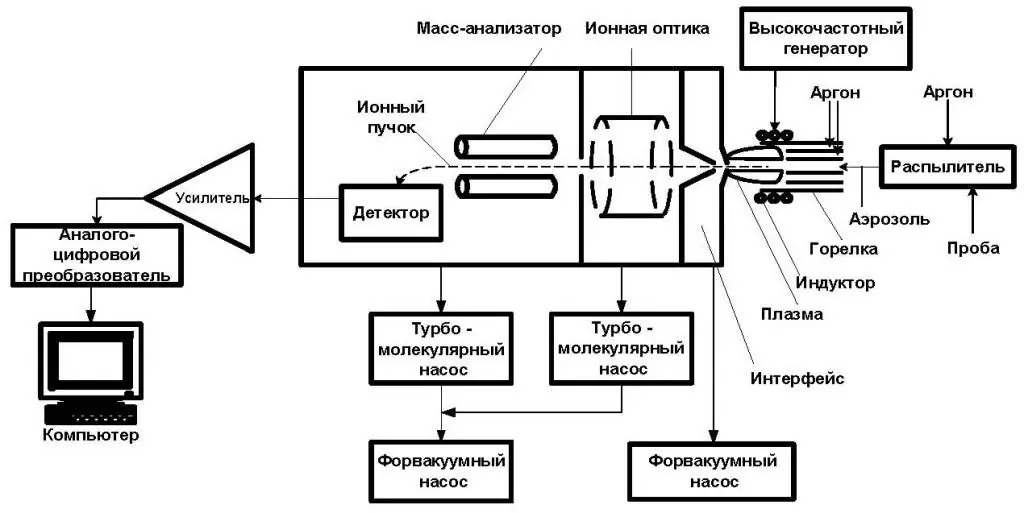
Spectral methods of analysis
A sample of the material (analyte) is introduced into the flame as a gas, a spray solution, or with a small loop of wire, usually platinum. The heat from the flame vaporizes the solvent and breaks chemical bonds, creating free atoms. Thermal energy also transforms the latter into excitedelectronic states that subsequently emit light when they return to their former form.
Each element emits light at a characteristic wavelength, which is scattered by a grating or prism and detected in a spectrometer. The trick most often used in this method is dissociation.
A common application for flame emission measurement is the regulation of alkali metals for pharmaceutical analytics. For this, the method of atomic emission spectral analysis is used.
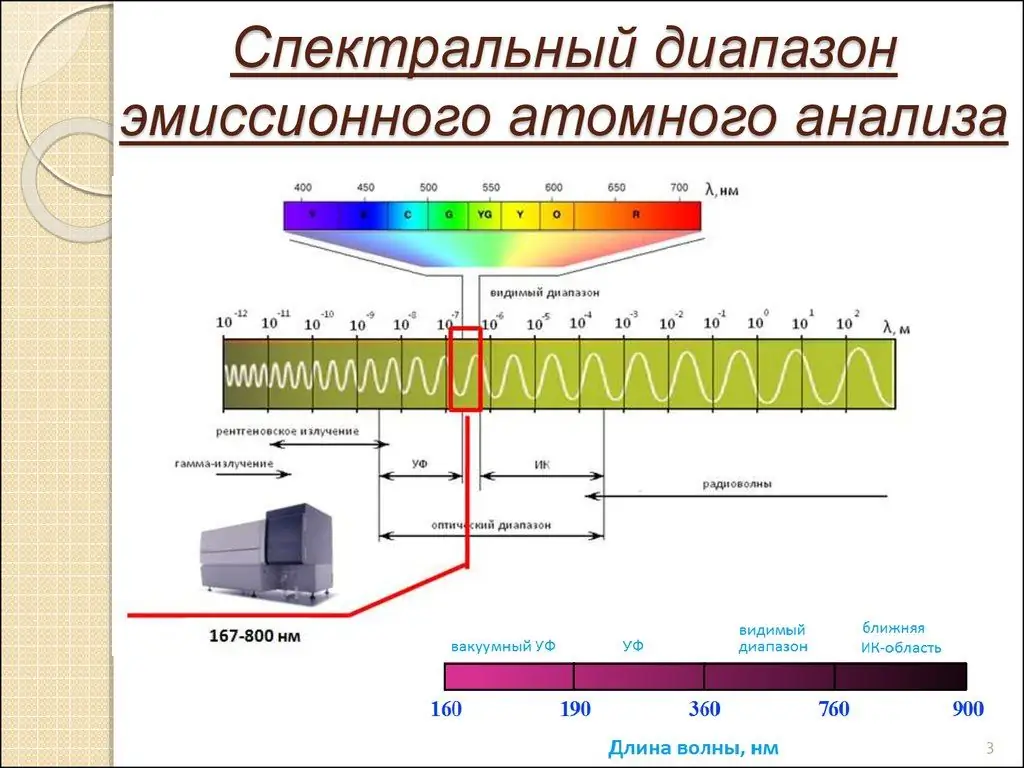
Inductively coupled plasma
Inductively coupled plasma atomic emission spectroscopy (ICP-AES), also called inductively coupled plasma optical emission spectrometry (ICP-OES), is an analytical technique used to detect chemical elements.
This is a type of emission spectroscopy that uses an inductively coupled plasma to generate excited atoms and ions that emit electromagnetic radiation at wavelengths characteristic of a particular element. This is a flame method with a temperature ranging from 6000 to 10000 K. The intensity of this radiation indicates the concentration of the element in the sample used in the application of the spectroscopic analysis method.
Main links and scheme
ICP-AES consists of two parts: ICP and optical spectrometer. The ICP torch consists of 3 concentric quartz glass tubes. The output or "working" coil of the radio frequency (RF) generator surrounds part of this quartz burner. Argon gas is commonly used to create plasma.
When the burner is turned on, a strong electromagnetic field is created inside the coil by a powerful RF signal flowing through it. This RF signal is generated by an RF generator, which is essentially a powerful radio transmitter that controls the "working coil" in the same way that a conventional radio transmitter controls a transmitting antenna.
Typical instruments operate at 27 or 40 MHz. The argon gas flowing through the burner is ignited by a Tesla unit, which creates a short discharge arc in the argon flow to initiate the ionization process. As soon as the plasma is "ignited", the Tesla unit turns off.
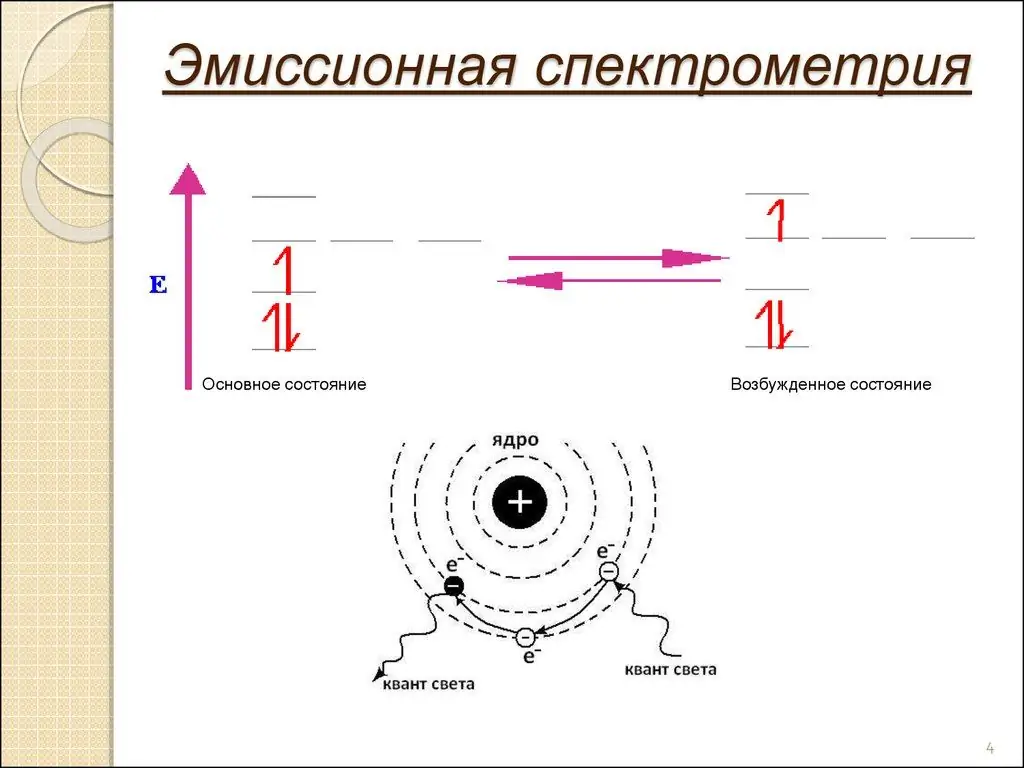
The role of gas
Argon gas is ionized in a strong electromagnetic field and flows through a special rotationally symmetrical pattern in the direction of the magnetic field of the RF coil. As a result of inelastic collisions created between neutral argon atoms and charged particles, a stable high-temperature plasma of about 7000 K is generated.
A perist altic pump delivers an aqueous or organic sample to an analytical nebulizer where it is converted into a mist and injected directly into the plasma flame. The sample immediately collides with electrons and charged ions in the plasma and itself decays into the latter. Various molecules split into their respective atoms, which then lose electrons and recombine repeatedly in the plasma, emitting radiation at the characteristic wavelengths of the elements involved.
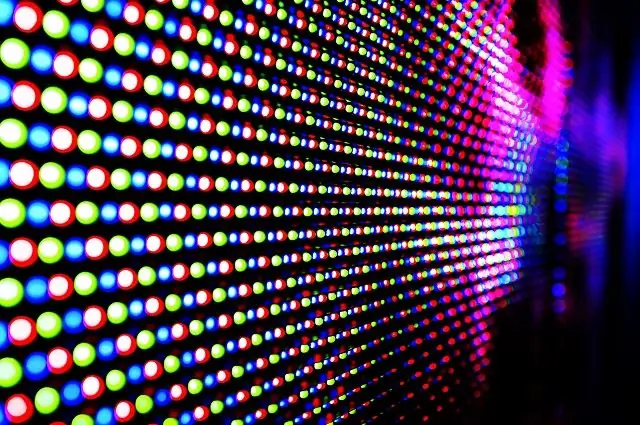
In some designs, a shear gas, usually nitrogen or dry compressed air, is used to "cut" the plasma at a specific location. One or two transmission lenses are then used to focus the emitted light onto a diffraction grating, where it is separated into its component wavelengths in an optical spectrometer.
In other designs, the plasma falls directly onto the optical interface, which consists of a hole from which a constant flow of argon exits, deflecting it and providing cooling. This allows emitted light from the plasma to enter the optical chamber.
Some designs use optical fibers to transmit some of the light to separate optical cameras.
Optical camera
In it, after dividing the light into its various wavelengths (colors), the intensity is measured using a photomultiplier tube or tubes physically positioned to "view" the specific wavelength(s) for each element line involved.
In more modern devices, the separated colors are applied to an array of semiconductor photodetectors such as charge-coupled devices (CCDs). In units using these detector arrays, the intensities of all wavelengths (within the range of the system) can be measured simultaneously, allowing the instrument to analyze every element to which the unit is currently sensitive. Thus, samples can be analyzed very quickly using atomic emission spectroscopy.
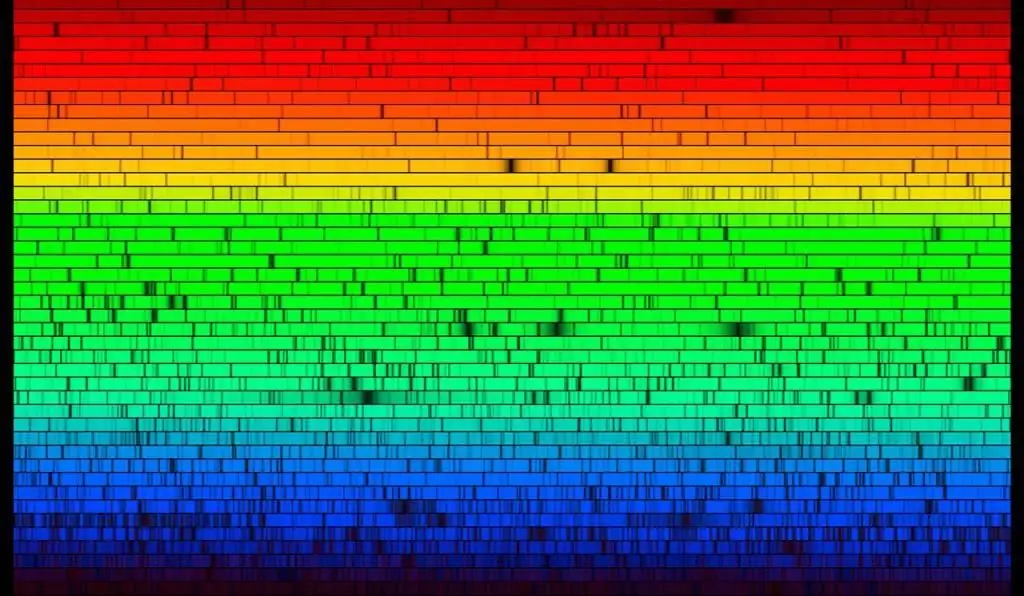
Further work
Then, after all of the above, the intensity of each line is compared with previously measured known concentrations of elements, and then their accumulation is calculated by interpolation along the calibration lines.
In addition, special software usually corrects for interference caused by the presence of various elements in a given matrix of samples.
Examples of ICP-AES applications include the detection of metals in wine, arsenic in foods, and trace elements associated with proteins.
ICP-OES is widely used in mineral processing to provide grade data for different streams to build weights.
In 2008, this method was used at the University of Liverpool to demonstrate that the Chi Rho amulet, found at Shepton Mallet and previously considered one of the earliest evidence of Christianity in England, only dates back to the nineteenth century.
Destination
ICP-AES is often used to analyze trace elements in soil and for this reason it is used in forensics to determine the origin of soil samples found at crime scenes or victims, etc. Although soil evidence may not be the only one in court, it certainly strengthens other evidence.
It is also rapidly becoming the analytical method of choice for determining nutrient levels in agricultural soils. This information is then used to calculate the amount of fertilizer needed to maximize yield and quality.
ICP-AESalso used for engine oil analysis. The result shows how the engine works. Parts that wear out in it will leave marks in the oil that can be detected with ICP-AES. ICP-AES analysis can help determine if parts are not working.
In addition, it is able to determine how much oil additives remain, and therefore indicate how much service life it has left. Oil analysis is often used by fleet managers or car enthusiasts who are interested in learning as much as possible about the performance of their engine.
ICP-AES is also used in the manufacture of motor oils (and other lubricants) for quality control and compliance with manufacturing and industry specifications.
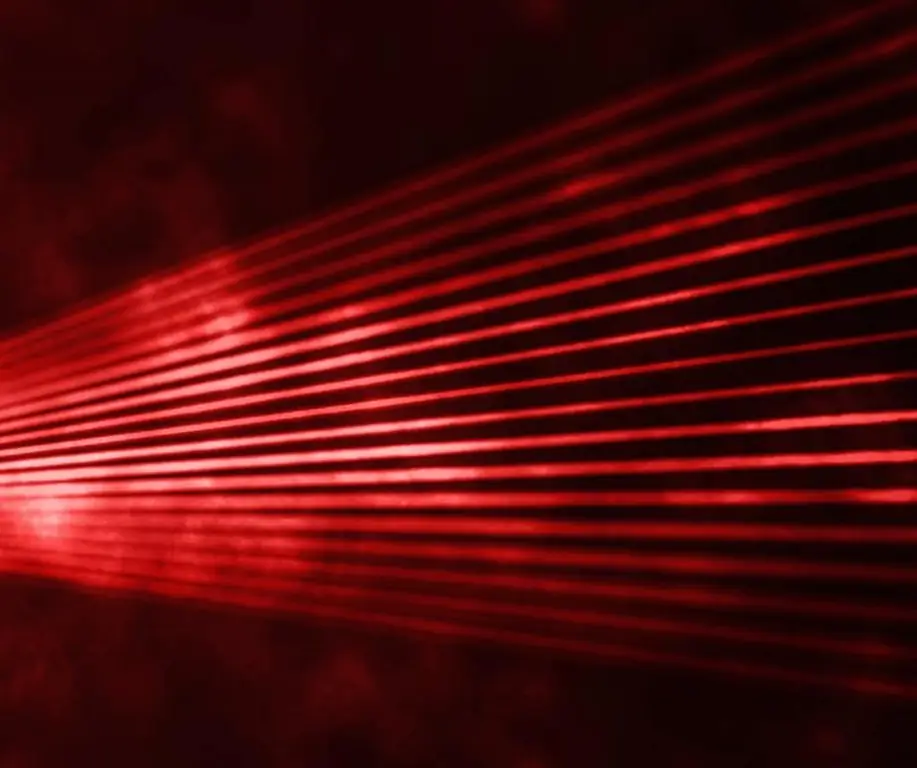
Another kind of atomic spectroscopy
Atomic absorption spectroscopy (AAS) is a spectral analytical procedure for the quantitative determination of chemical elements using the absorption of optical radiation (light) by free atoms in the gaseous state. It is based on the absorption of light by free metal ions.
In analytical chemistry, a method is used to determine the concentration of a particular element (an analyte) in an analyzed sample. AAS can be used to determine more than 70 different elements in solution or directly in solid samples through electrothermal evaporation, and is used in pharmacological, biophysical and toxicological research.
Atomic absorption spectroscopy for the first timewas used as an analytical method in the early 19th century, and the underlying principles were established in the latter half by Robert Wilhelm Bunsen and Gustav Robert Kirchhoff, professors at the University of Heidelberg, Germany.
History
The modern form of AAS was largely developed in the 1950s by a group of Australian chemists. They were led by Sir Alan Walsh of the Commonwe alth Scientific and Industrial Research Organization (CSIRO), Division of Chemical Physics, in Melbourne, Australia.
Atomic absorption spectrometry has many applications in various fields of chemistry such as the clinical analysis of metals in biological fluids and tissues such as whole blood, plasma, urine, saliva, brain tissue, liver, hair, muscle tissue, semen, in some pharmaceutical manufacturing processes: minute amounts of catalyst remaining in the final drug product and water analysis for metal content.
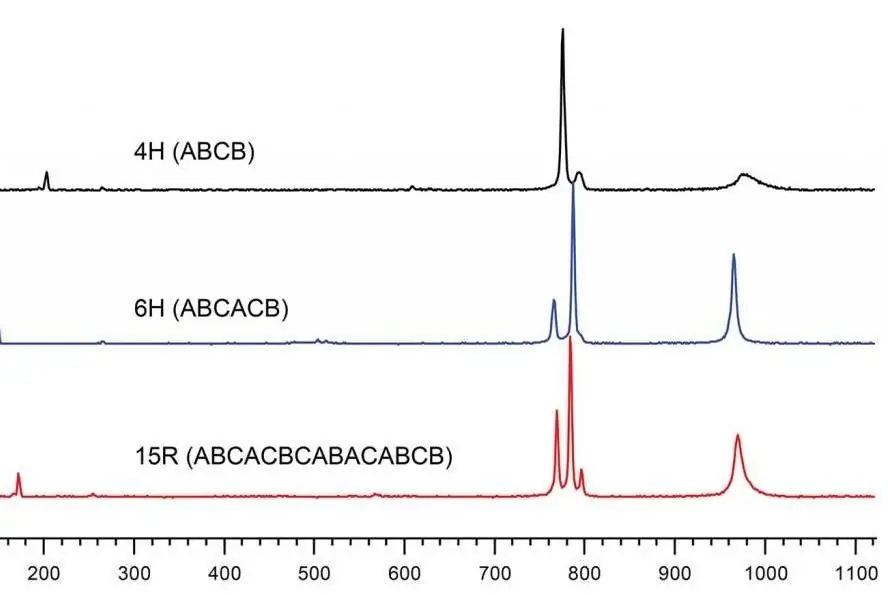
Scheme of work
The technique uses the atomic absorption spectrum of a sample to estimate the concentration of certain analytes in it. It requires standards with known constituent contents to establish a relationship between measured absorbance and their concentration, and is therefore based on the Beer-Lambert law. The basic principles of atomic emission spectroscopy are exactly as listed above in the article.
In short, the electrons of the atoms in the atomizer can be transferred to higher orbitals (excited state) in a shortperiod of time (nanoseconds) by absorbing a certain amount of energy (radiation of a given wavelength).
This absorption parameter is specific to a particular electronic transition in a particular element. As a rule, each wavelength corresponds to only one element, and the absorption line width is only a few picometers (pm), which makes the technique elementally selective. The scheme of atomic emission spectroscopy is very similar to this one.